Transcriber’s note:
The cover image was created by the transcriber and is placed in the public domain.
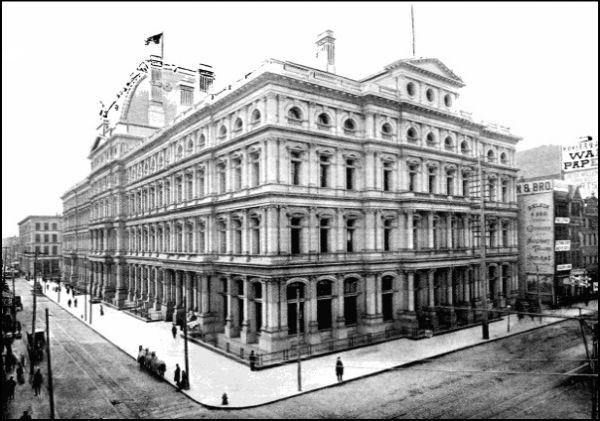
THE MAIN POST-OFFICE, PHILADELPHIA.
The
Pneumatic Despatch Tube System
of the
Batcheller Pneumatic Tube Co.
ALSO
FACTS AND GENERAL INFORMATION
RELATING TO PNEUMATIC
DESPATCH TUBES
BY
B. C. BATCHELLER, B.Sc.
MECHANICAL ENGINEER
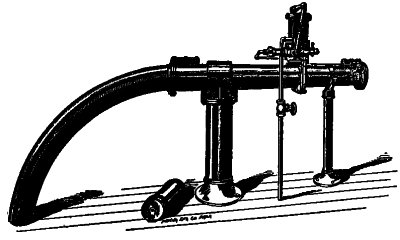
PHILADELPHIA
PRESS OF J. B. LIPPINCOTT COMPANY
1897
Copyright,
1896,
by
B. C. Batcheller.
CHAPTER I. | |
---|---|
A BRIEF HISTORICAL SKETCH. |
PAGE |
Early Records |
9 |
Practical beginning of the Art—The London Pneumatic Telegraph |
10 |
The Siemens Circuit System |
14 |
Recent Improvements in the London System |
16 |
An Underground Pneumatic Railway for Transportation of Mail | 19 |
The Berlin Pneumatic Telegraph |
20 |
The Paris Pneumatic Telegraph |
22 |
The Pneumatic Telegraph of other Cities |
25 |
Pneumatic Tubes in America |
25 |
CHAPTER II. | |
THE PNEUMATIC TRANSIT COMPANY AND THE FIRST PNEUMATIC TUBES FOR THE TRANSPORTATION OF UNITED STATES MAIL. |
|
Organization |
28 |
Aim and Object of the Company |
28 |
The Clay-Lieb Patents |
30 |
Franchises and First Government Contract |
33 |
Search for Tubes |
34 |
Method of Manufacturing Tubes |
35 |
Laying and Opening the Tubes for Traffic |
37 |
4 Description of the Tubes, Method of Laying, etc. |
38 |
The Air-Compressor—Method of Circulating the Air |
40 |
Terminal Apparatus |
42 |
The Sender |
43 |
Sub-Post-Office Receiver |
44 |
Main Post-Office Receiver |
47 |
The Carrier |
50 |
Operation of the Tubes |
52 |
Benefits of the System |
54 |
CHAPTER III. | |
THE SYSTEM AND APPARATUS OF THE BATCHELLER PNEUMATIC TUBE COMPANY. |
|
General Arrangement and Adaptability of the System |
57 |
The Size of Tubes |
64 |
System of very Large Tubes |
65 |
General Arrangement of Apparatus in the Stations—Two-Station, Two-Compressor Line |
69 |
Two-Station, One-Compressor Line |
72 |
Three- to Eight-Station Line |
74 |
Sending Apparatus |
79 |
Sending Time-Lock |
84 |
Intermediate Station Time-Lock |
88 |
Electro-Pneumatic Circuit-Closer |
91 |
The Open Receiver |
94 |
The Closed Receiver |
99 |
The Intermediate Station Receiving and Transfer Apparatus |
106 |
Carriers |
115 |
Air Supply |
117 |
Fans |
117 |
Blowers |
117 |
Air Compressors |
118 |
5 The Tube, Line Construction, etc. |
122 |
CHAPTER IV. | |
FACTS AND GENERAL INFORMATION RELATING TO PNEUMATIC TUBES. |
|
Definitions |
124 |
Intermittent and Constant Air-Current |
125 |
Laws Governing the Flow of Air in Long Tubes |
126 |
Law of Pressure |
128 |
Uses of Pressure Curves |
130 |
Law of Velocity |
130 |
Characteristics of the Velocity Curve |
132 |
Uses of Velocity Curves |
133 |
Quantity of Air Used |
134 |
Temperature of the Air |
135 |
Horse-Power |
136 |
Efficiency |
137 |
Pressure and Exhaust Systems |
138 |
Laws expressed in Mathematical Formulæ |
141 |
Moisture in the Tubes |
142 |
Location of Obstructions in Tubes |
143 |
PREFACE.
I have been prompted to prepare this book by the frequent inquiries made regarding the details of our system of pneumatic tubes. These inquiries have come from people interested in our company, from others interested in companies that have purchased the right to use our apparatus, from people desirous of becoming interested in a pneumatic-tube business, from would-be purchasers of pneumatic tubes, and from people interested in pneumatic tubes from a scientific, engineering, or mechanical point of view. This book is not intended to be a treatise on pneumatic tubes. In the first chapter I have given a brief sketch of what has been done in the application of pneumatic tubes from the earliest records to the present time. The second chapter contains a description of the postal tubes in Philadelphia, and the third chapter describes our system in detail. Following this is a short chapter explaining the theory of pneumatic tubes, or the theory of the flow of air in long pipes, stating the more interesting facts and relations in as plain and simple a8 manner as possible. Mathematical formulæ have been purposely avoided.
Several plates showing the Philadelphia postal line have been kindly loaned to me by the Engineers’ Club of Philadelphia. They formerly appeared in a paper read by Mr. A. Falkenau before that club. I have also to thank the and Drill Co., the B. F. Sturtevant Co., the Wilbraham-Baker Blower Co., and J. B. Stewart for the use of electrotypes of their machines.
B. C. B.
October 6, 1896.
THE
Batcheller Pneumatic Tube System.
————————
CHAPTER I.
A BRIEF HISTORICAL SKETCH.
Early Records.
—The earliest reference to pneumatic transmission of which we find any record is a paper presented to the Royal Society of London, by Denis Papin, in the year 1667, entitled “Double Pneumatic Pump.” His plan was to exhaust the air from a long metal tube by two large cylinders. The tube was to contain a piston, to which a carriage was attached by means of a cord. The “American Cyclopædia” goes on to say, “More than a century elapsed before any further effort in this direction was made. Paucbrouke’s ‘Dictionnaire Encyclopédique des Amusements des Sciences’ (1792) gives a description of a machine by M. Van Estin, by means of which a hollow ball holding a small package was propelled by a blast of air through a tube several hundred feet in length, and having many curves. This plan seems, however, to have been more an amusement than an attempt to introduce an industrial scheme. With more regard to practical results, Medhurst, an engineer of London, published a pamphlet on the subject in 1810. He proposed to move10 small carriages on rails in air-tight tubes or tunnels, by compressed air behind, or by creating a partial vacuum in front. In 1812 he published another pamphlet; but the plan was not put into successful operation, principally from insufficient means of exhaustion. About 1832 he proposed to connect the carriage inside such a tube with a passenger carriage running on the top of the tube; and, although the latter project has never been commercially successful, it was the first to be practically attempted. More than a score of patents were taken out on the Continent and in England and America, none of which met with any practical success. Returning to the original idea of Denis Papin, inventors attempted to accomplish pneumatic transmission by moving the load inside the tube, and in course of time achieved success. In France MM. Jarroux and Taisseau presented a project for atmospheric telegraphy before the Academy of Sciences, and they were succeeded in the same direction by MM. Brochet and Ardor.”
Practical Beginning of the Art. The London Pneumatic Telegraph.
—London has the honor of being the first city to have a practical system of pneumatic telegraphy. The first tubes were installed by the Electric and International Telegraph Company, the work being planned and carried out by their engineer, Mr. Josiah Latimer Clark, in 1853 and 1854. The first tube to be laid was one and one-half inches in diameter, and extended from the central station, Founder’s Court, Lothbury, to the Stock Exchange, Throgmorton Street, a distance of two hundred and twenty yards. The tube was operated intermittently by connecting it to a vacuum chamber at the11 central station. Carriers were sent only in one direction. A steam-pump was used to maintain the vacuum. Much experience was gained from the use of this first tube. In 1858 some improvements were made by Mr. C. F. Varley, and I can best describe them by quoting from the discussion of Mr. Carl Siemens’s paper on “Pneumatic Despatch Tubes: The Circuit System” before the Institution of Civil Engineers, as recorded in the minutes of that society. “Later, about the year 1858, when a pipe two and one-fourth inches internal diameter was extended from Telegraph Street to Mincing Lane, thirteen hundred and forty yards in length, the traffic was so considerable that it was found desirable to have the power of sending messages in both directions. To effect that a smaller pipe, one and one-half inches in internal diameter, was laid between Telegraph Street and Mincing Lane, with a view to carrying the vacuum to the latter station, so as to take messages in the opposite direction. This smaller pipe was found to so wiredraw the current that the pipe would not work, the leakage past the carrier being too considerable; and accordingly a large chamber was built in the basement floor or kitchen at the corner of Mincing Lane and Leadenhall Street to collect power or vacuum for bringing the messages from Telegraph Street to Mincing Lane. This chamber was constructed of timber, fourteen feet by twelve feet broad and ten feet high, and was covered with lead. It was not strong enough to withstand the pressure; for one day, a carrier having stuck half-way, and when there was a higher vacuum than usual,—viz., twenty-three inches of mercury,—it collapsed with a loud report. At the time12 the landlord of the house happened to be dining in the next room, and he suddenly found himself, his table, dinner, and the door, which was wrenched off its hinges, precipitated into the room amongst the débris of the chamber. The windows were forced inwards, and those on the opposite side of Mincing Lane and Leadenhall Street were drawn outwards. The damage was considerable. This accident put an end, for a time, to the attempt to send telegraph messages by means of a vacuum conveyed through this smaller pipe. About that time he (Mr. Varley) became the engineer-in-chief of the Electric Telegraph Company, and got permission from the directors to introduce a new system,—viz., compressed air,—though many persons contended that it would be impossible to blow messages through a pipe, because all attempts to blow air through long pipes had utterly failed; while others said that, if messages were sent, they would go much slower than with the vacuum.... In his (Mr. Varley’s) apparatus, for he was the first to introduce compressed air, the reverse was found to be the case, and for this reason: the tube did not consume power until a message was about to be forwarded; and in a tube thirteen hundred yards in length, and two and one-fourth inches in diameter, fifteen seconds elapsed before the vacuum was felt at the distant end after communication had been established with the exhausted chamber at the engine end of the tube, consequently the carrier did not start until after fifteen seconds had elapsed. When a message was sent by compressed air, it was sent from the end at which the power was applied, and the carrier started at once, thus gaining a start of fifteen13 seconds; now, inasmuch as the air in the tube had to be compressed, it started at a very high velocity, and when it reached the other end the compressed air in expanding gave it a higher velocity. The result was, in thirteen hundred and forty yards, from Telegraph Street to Mincing Lane, the carriers were drawn by vacuum, on an average, in from sixty to seventy seconds, and were propelled by compressed air in about fifty or fifty-five seconds, the difference of pressure in each case being nearly equal.”
The first one and one-half inch tubes laid under the direction of Mr. Clark were of iron with screwed joints. They gave much trouble from roughness upon the interior, which wore the carriers very rapidly, and from water that was drawn in through leaky joints. When the extensions were made in 1858 and afterwards, two and one-fourth inch lead tubes were used with plumber’s joints made over a heated mandrel, which made the joints very smooth upon the interior. The carriers were of gutta percha in the form of a cylinder closed at one end and fitted with a cap at the other. The outside was covered with felt or drugget.
When a carrier was to be despatched, a signal was sent to an attendant at the pump end of the tube, who closed that end and connected the tube to an exhausted chamber by opening a valve. As soon as the carrier arrived, he closed the valve and opened the tube, which allowed the carrier to drop out. Mr. Varley improved the method of operating the valves by making the air pressure do the work by means of cylinders and pistons when the attendant pressed a button. He also improved the carriers by doing14 away with the cap and using in its place an elastic band to hold the messages in place.
We have seen that Mr. Clark designed the first tube used in connection with the telegraph, and that it was a single tube, operated in one direction only by vacuum, being operated only when there were messages to send. This was extended and improved by Mr. Varley, who increased the diameter of the tubes from one and one-half inches to two and one-quarter inches, and operated them in both directions, using vacuum for sending in one direction and compressed air for sending in the other. The air current was maintained in the tubes only when messages were sent.
Great credit is due to Sir Rowland Hill, who, in 1855, had a proposed method of conveying mails in the city of London, through nine- and thirteen-inch tubes, thoroughly investigated. It was decided at this time that the saving in time over that consumed by mail carts would not warrant the expense of installing such a system.
The Siemens Circuit System.
—The next progressive step was made by Siemens Brothers, of Berlin, who proposed a new system called the “circuit system,” in which two tubes were used, the up tube being connected to the down tube at the distant end. The air was compressed into one end of the circuit and exhausted from the other, and, furthermore, it was kept in constant circulation. Carriers were despatched by inserting them into the air-current without stopping it, in one direction in one tube or in the opposite direction in the other. Another feature of the Siemens system was the placing of three or more15 stations on one double line of tubes. Carriers could be stopped at an intermediate station by inserting in the tube an obstructing screen which the air would pass but which would stop a carrier. This system is described in detail in a paper read by Mr. Carl Siemens before the Institution of Civil Engineers, London, November 14, 1871, Vol. XXXIII. of the Proceedings. The Siemens apparatus for sending and receiving carriers consisted of two short sections of tube attached to a rocking frame so that either could be swung by hand into line with the main tube. One of the tube sections was open at both ends, and was used for despatching carriers. A carrier was placed in it, then it was swung into line with the main tube, when the air-current passing through swept the carrier along. The other tube section contained a perforated screen in one end and was used to receive carriers. When it was in line with the main tube and a carrier arrived, the carrier was stopped by striking the screen, then the tube section was swung to one side and the carrier pushed out with a rod. A by-pass was provided for the air around the apparatus so that its flow was not checked when the tube section was swung. When a carrier was despatched to an intermediate station, a signal was sent, and then the section of tube containing the screen was interposed in the line of the tube to stop the carrier upon its arrival. The carriers used by Mr. Siemens were made of gutta-percha, papier maché, or tin, closed at one end and fitted with a cover at the other. They were covered with felt, drugget, or leather. The front ends of the carriers were provided with thick disks of drugget or16 leather fitting the tube loosely, and the opposite ends were surrounded with pieces of the same material attached to them like the leather of an ordinary lifting pump.
In 1869 Messrs. Siemens Bros. received an order from the British government to install an experimental line of tubes between the central telegraph station and the general post-office. This was completed in 1870, and after a half-year’s test it was extended to Fleet Street, and finally to Charing Cross. The tubes were of iron, three inches in diameter, with flanged and bolted joints. It was found, after some experience, that there was no advantage in the circuit, so the up and down tubes were separated at Charing Cross Station and worked independently.
Recent Improvements in the London System.
—In 1870 Mr. J. W. Wilmot designed a double sluice-valve by means of which carriers could be despatched continuously without stopping the flow of air in the tubes. Mr. Wilmot further increased the working capacity of pneumatic tubes when, in 1880, he invented an intermediate automatic signaller, by means of which a carrier signals the passage of a given point on its journey, showing that the section of the tube traversed is clear, thus allowing a second carrier to be despatched before the first has reached its destination.
From this beginning the English system developed into what has been termed a “radial system;” that is to say, one principal and several minor central pumping stations have been established, and from these radiate tubes to numerous sub-stations (see Fig. 2). Some of the stations are connected with double lines for sending in opposite directions. The out-going tube from the pumping station is worked17 by compressed air, and the incoming tube by exhaustion. Other stations are connected by single tubes, and they are operated alternately by compression and exhaustion. Intermediate stations are located on some of the lines. For the central station the Varley valves were found too expensive and troublesome to keep in order, so they were replaced by the Wilmot double sluice-valves, which are operated manually. In recent years the sluice-valves have been in turn replaced by what are termed D-boxes, a simpler form of apparatus. At the sub-stations the tube terminates in a box into which the carriers drop. As the system has been gradually extended, tubes two and three-sixteenths inches inside diameter have been used for short lines, and three-inch tubes for long lines. The tubes are of lead laid inside a cast-iron pipe which serves as a shield, protecting them from injury. They are laid in twenty-nine18 foot sections, the joints being made by soldering over a steel mandrel, which is afterwards drawn out by a chain. The joints in the cast-iron protecting pipe are made by caulking with yarn and lead. “Electric signals are used between the central and sub-stations, consisting of a single stroke bell and indicator, giving notice of the arrival and departure of carriers, and to answer the necessary questions required in working. Where there are intermediate stations the tubes are worked on the block system, as if it were a railway. Experience shows that, where great exactness of manipulation cannot be obtained, it is necessary to allow only one train in each section of a tube, whether worked by vacuum or pressure. But where there is no intermediate station, and where the tube can be carefully worked, carriers may be allowed to follow one another at short intervals in a tube worked by vacuum, although it is not perfectly safe to do so in one worked by pressure. In working by pressure it has been found that, notwithstanding a fair interval may be allowed, carriers are apt to overtake one another, for no two carriers travel in the same times, because of differences in fit, unless they are placed end to end. If signalling be neglected and a carrier happens to stick fast, being followed by several others, a block will ensue which it will be difficult to clear, while the single carrier could readily have been dislodged.” (Proceedings Institute of Civil Engineers, London, Vol. XLIII. p. 61.)
No changes have been made in the carriers from those used in the early experiments which have already been described.
The London system has grown until it now includes no less than forty-two stations and thirty-four miles of tubes. Similar systems have been established in connection with the telegraph in Liverpool, Manchester, Birmingham, Glasgow, Dublin, and New Castle. The tubes give a cheaper and more rapid means of despatching telegrams between sub-stations and central stations than transmission by telegraph, and local telegrams can be delivered in the sender’s handwriting.
An Underground Pneumatic Railway for Transportation of Mail.
—Before describing the systems used in the cities on the Continent of Europe, we must notice a very large pneumatic tube, or more properly called a pneumatic tunnel railway, constructed in London for the transportation of mail from one of the railway stations. The first railway of this type was constructed in 1863 by the Pneumatic Despatch Company of London, and extended from Euston to the district post-office in Eversholt Street, a distance of about eighteen hundred feet. The tunnel was flat on the bottom, having a D-shaped cross-section two feet eight inches by two feet eight inches. The carriers or carriages were cradle-like boxes fitting the tunnel, and they moved at a speed of seventeen miles per hour, carrying fifteen mails daily. In 1872 two similar but larger tunnels were built from Euston Station to the general post-office, a distance of fourteen thousand two hundred and four feet, or two and three-quarters miles. One was for the up traffic, and the other for the down. The tunnels were four and a half feet wide by four feet high, the straight part being built of cast iron and the20 bends of brick. The line was operated by a fan twenty-two feet in diameter, which forced the air into one tunnel and exhausted it from the other, producing a vacuum of ten inches of water, or six ounces per square inch. The carriages occupied twelve minutes in traversing the tunnels, and there was one gradient of one to fourteen. The carriages were ten feet four inches long and weighed twenty-two hundredweight. “The system was able to transport over the whole line, allowing for delays, an average of a ton per minute.” The system was used to transport the mails in bulk, but it was found to be slow and unsatisfactory, and was very soon abandoned.
The Berlin Pneumatic Telegraph.
—In 1863 the Prussian government applied to the firm of Siemens and Halske, of Berlin, for a proposition to establish a system of pneumatic tubes in that city for the transmission of telegraph messages. A proposition was accordingly submitted, and the work was completed in 1865. This first line consisted of two parallel wrought-iron tubes, two and one-half inches in diameter, one tube being used exclusively to send in one direction, and the other in the opposite direction. They extended from the telegraph station to the Exchange, requiring a total length of five thousand six hundred and seventy feet of tube. The two tubes were looped together at the Exchange, and a continuous current of air was made to circulate in them by a double-acting steam air-pump, located at the telegraph station. Air was compressed into one end of the tube and exhausted from the other. With nine inches of mercury pressure and vacuum the passage was made in ninety-five seconds to the Exchange, and21 seventy-five seconds from the Exchange. It was similar to the line established in London by the same firm some years later, which we have already described, except that there was no intermediate station. After the line had been in use for a year and a half, the Prussian government had it extended, first, from the telegraph station to the Potsdam gate, with an intermediate station at the Brandenburg gate. After these preliminary experiments, further extensions were made until a net-work of tubes extended over the city of Berlin, including no less than thirty-eight stations and over twenty-eight miles of tubes; but in laying down this net-work a departure was made from the Siemens system. Air was no longer kept constantly circulating, but power was stored up in large tanks, some being exhausted and others filled with compressed air, which was used when required to send messages, usually at intervals of five or fifteen minutes. The exhausted tanks were permanently connected with the closed tubes, which were opened when required for use. The tanks containing compressed air were connected to the tubes when messages were sent. The internal diameter of the tubes was 2.559 inches. They were laid in circuits, including several stations in a circuit, and the carriers travelled only in one direction around the circuit. Some outlying stations were connected by a single tube with central pumping-stations, these single tubes being worked in both directions. Years of experience have shown the disadvantages of this circuit-system, and it has gradually been changed to the radial system, such as is used in London, until now nearly all the stations are grouped around the central pumping-stations, to which they are connected22 directly by radiating tubes. The Siemens apparatus has been replaced by simpler and less expensive valves and receiving-boxes, the latest form of which was designed and patented by Mr. Josef Wildemann.
The Paris Pneumatic Telegraph.
—We will now glance at the system used in Paris, which has some novel features. In 1865 it was decided to establish a system, and the first experimental line, from Place de la Bourse to the Grand Hôtel, on the Boulevard des Capuciens, was laid in 1866. Instead of using a steam-engine to drive an air-compressor or exhaust-pump, air was compressed in tanks by displacement with water from the city mains. In 1867 this line was extended to Rue de Grennelle St. Germain, with an intermediate station at the Rue Boissy d’Anglais, and another line with stations at Rue Jean Jacques Rousseau, Hôtel du Louvre in the Rue de Rivoli, the Rue des Saints Pères, and terminating in the central station. In 1868 the system was changed to a polygonal or circuit system by removing the station in the Rue de Rivoli to the Place du Théâtre Français and connecting the latter directly with the Bourse. Other changes and extensions were made in 1870 and 1871, until three polygons or circuits were formed, with five or six stations in each circuit, and several outlying stations were connected by independent tubes. In the middle of the year 1875 seventeen stations had been connected and plans were made for twenty-one more. Instead of maintaining an air-current around each circuit by machinery located at one of the stations on the circuit, at least three of the five or six stations comprised in the circuit have means of supplying compressed air or23 of exhausting it, and each side of the polygon, or section of the circuit between two stations, is operated independently of the rest of the circuit (see Fig. 3). Carriers are sent on from station to station around the circuit, either by compressed air from the last station from which they were sent or by means of exhaustion at the next station towards24 which they are moving. The carriers are made up in trains of from six to ten, with a piston behind them that fits the tube closely and forces them ahead. Each carrier is addressed by means of a label for its destined station. Trains are despatched around the circuits at stated times, usually at fifteen-minute intervals. As they arrive at the various stations, carriers are taken out and others put in, and the trains sent on their way. The carriers consist of iron cylinders, closed at one end, with a leather case that slides over them and closes the open end. They weigh, when filled with thirty-five messages, twelve and one-half ounces, and they will travel about twelve hundred miles before the leather cover is so worn that it must be thrown away. The pistons are made of a wooden cone, covered with iron, and having a “cup-leather” upon the rear end that fits the tube closely. The sending and receiving apparatus consists of sections of tube closed at one end, having a door on the side, through which carriers are inserted or despatched. A peculiar form of fork is used for picking them out. The air is controlled by valves opened and closed by hand.
Several methods are used to compress and exhaust the air. The most novel method consists in having tanks in which a partial vacuum is produced by allowing water to flow out of them into the sewer, or in having the air compressed by allowing water from the city mains to flow into the tanks and displace the air. Water jets have also been used, operating similar to a steam-injector. At some of the stations water turbines drive the air-pumps, and at others steam-engines are used.
The tubes of the Paris system are of wrought iron, in lengths of from fifteen to twenty feet, the joints being made with flanges and bolts. The interior diameter is 2.559 inches with a maximum variation to 2.519 inches. The bends are made with a radius of from six to one hundred and fifty feet. Water frequently gives trouble by accumulating in the tubes. Traps are placed at low points to drain it off.
The speed of the trains of carriers in the Paris tubes is from fifteen to twenty-three miles per hour, and the average time that elapses from the receipt of a message until its delivery is from forty to forty-five minutes.
The Pneumatic Telegraph of other Cities.
—A system similar to the one just described is used in Vienna. It differs some in details of apparatus, but the carriers are despatched around circuits in trains, stopping at each station, where some carriers are removed and others inserted. Brussels also is not without its system of pneumatic tubes for the transmission of telegrams.
Pneumatic Tubes in America.
—Turning our attention now to our own country, we cannot pass without mention some experiments of Alfred E. Beach with pneumatic railways, made nearly thirty years ago. His first experiment upon a large scale was made at the American Institute Fair held in New York City in 1867. Here he had constructed a circular wooden tube, one hundred and seven feet long and six feet in diameter. A car that would seat ten people ran upon a track laid down inside the tube, and was propelled by a helix fan ten feet in diameter, making two hundred revolutions per minute.26 He next tried his railway upon a practical scale, constructing an eight-foot tunnel for two hundred feet under Broadway, starting at the corner of Warren Street. A car was propelled by a large rotary blower located in the basement of a building near by. The blower was kept constantly running, and the car was sent alternately in one direction and then the other by changing valves at the blower. Few people know that this experimental line still exists under Broadway as Mr. Beach left it.
The most extensive use of small pneumatic tubes in this country has been in our large retail department stores for despatching cash to and from a centrally located cashier’s desk. Seamless brass tubes are usually used, and, since the tubes are short, the air is either compressed or exhausted by means of positive rotary blowers. At the outlying stations the tubes are usually open to the atmosphere, while at the central station simple forms of valves are used for sending and receiving. An outgoing and a return tube are always used, and the air is kept in constant circulation. The carriers are of metal with felt packing rings and open on the side. These cash-carrying systems have come into use during the past twenty-five years.
The Western Union Telegraph Company uses small tubes to transmit its messages to a considerable extent in some of our large cities. In 1876 four lines were laid in New York City from the main office on Broadway: two to the branch office at No. 14 Broad Street, one to 134 Pearl Street, and one to the Cotton Exchange. Since then this company has laid a double line about two miles in length under Broadway to its up-town office. It also uses27 tubes to send messages from the receiving desks to the operating rooms within the buildings.
Many of our large hotels use pneumatic tubes to transmit messages to the different floors and offices of the buildings, taking the place of messenger, or bell boys, who formerly did this service.
We call especial attention to the fact that in all the systems that we have mentioned which are in use both in this country and in Europe, none of the tubes are larger than three inches internal diameter; also that in all the systems, except in Paris where the carriers are despatched in trains, the carriers are so light and move so slowly that they can be stopped by allowing them to come in contact with some solid object, such as a box into which the carriers drop. Very few of the tubes are more than two miles in length, and most of them are less than one mile. A speed of more than thirty miles per hour has seldom been attempted, and usually it is much less than this.
CHAPTER II.
THE PNEUMATIC TRANSIT COMPANY AND THE FIRST PNEUMATIC TUBES FOR THE
TRANSPORTATION OF UNITED STATES MAIL.
Organization.
—Early in the year 1892 several Philadelphia gentlemen organized a corporation and obtained a charter in the State of New Jersey to construct, lay, and operate pneumatic tubes for the transmission of United States mail, packages, merchandise, messages, etc., within the States of New Jersey and Pennsylvania. The corporation was styled the Pneumatic Transit Company. Mr. William J. Kelly was elected president, and the company is still under his management.
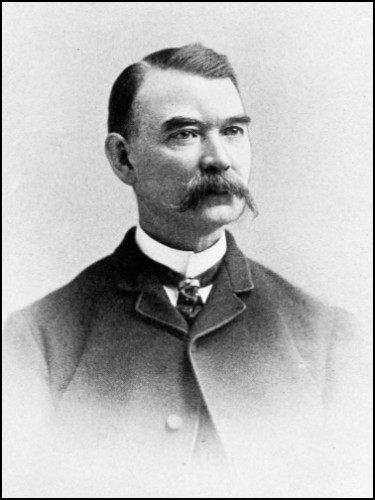
WM. J. KELLY,
President of the Pneumatic Transit Co.
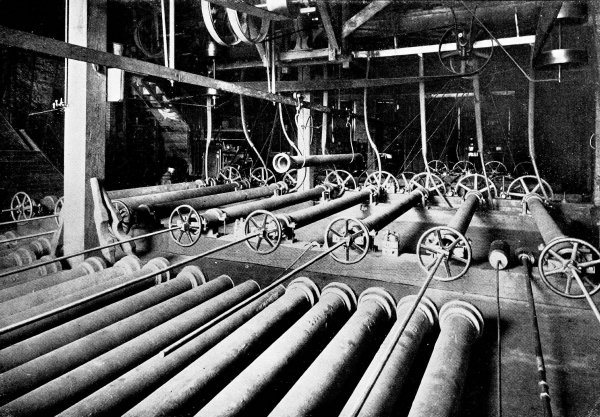
Fig. 4.
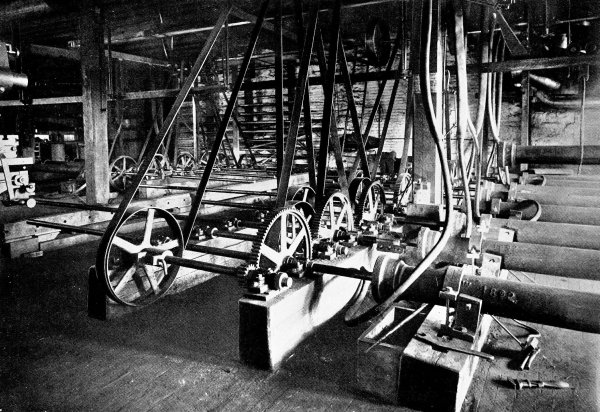
Fig. 5.
SIX-INCH PNEUMATIC TUBES IN PROCESS OF BORING AT THE SHOP OF A.
FALKENAU, PHILADELPHIA, PA.
Aim and Object of the Company.
—When the Pneumatic Transit Company was formed, it was the aim and object of its promoters to construct an extensive system of underground tubes in the City of Philadelphia which would serve, first, for the rapid transmission of mail, second, for the quick delivery of merchandise from the large retail stores, third, for the transmission of telegrams or messages within the city limits, and, fourth, to conduct a general local express business with greater speed than can be done in any other manner. To accomplish this result sub-stations were to be located six or eight blocks apart throughout a large portion of the city, and a central station was to be established in the centre of the business29 section. Stations were also to be established in the more important retail stores and large office buildings, and all of the stations were to be connected by tubes forming one large system.
For the transmission of mail it was planned to connect the main post-office with the sub-post-offices by tubes of a size large enough to carry all of the first-class and most of the other classes of mail matter. The sub-post-offices would be divided into groups, all of the offices in one group being connected to the same line, which would terminate at the main post-office. Most of the business would be between the main and individual sub-offices; in addition to this there would be some local mail sent between the sub-offices which, for offices in the same group, could be despatched directly without passing through the main office. The advantages to be gained by the use of these tubes over the present wagon service are very apparent. It places all the sub-post-offices in almost instant communication with the main office and with each other.
It was a part of the general plan to lay tubes from the main post-office to the railway stations, thereby hastening the despatch and receipt of mails to and from the trains.
It was expected that the bulk of the business would consist in the delivery of parcels from the retail stores to the private houses in the residence sections of the city. Of course it would not be practicable to lay a tube to each house, but with a station not more than four or five blocks away, the parcels would be sent through the tube to the nearest station, and then delivered by messengers to the houses with a minimum loss of time. Ladies could do their30 shopping and find their purchases at home when they returned.
The same tubes used for parcel delivery would also be used for a district messenger service. With numerous public stations in convenient locations, all the advantages of the European system would be realized in the quick despatch of letters and telegrams. Every one knows how much time is consumed by district messenger-boys in the delivery of messages, especially when they have to go long distances, and no argument is required to show that this time would be very much reduced by the use of pneumatic tubes, besides prompt delivery would be made much more certain.
The tubes of this system were to be six or eight inches in diameter, with a few small tubes in localities where the message service is very heavy.
Without going more into detail, such were in brief the plans of the promoters of this new company; but before launching such an enterprise, involving a large amount of capital, there were many engineering and mechanical problems to be solved. It was not simply a question of obtaining tubes and laying them in the streets, but ways and means for operating them must be devised. Up to this time only small tubes had been used for the transmission of telegrams, messages, cash, and other light objects. Now it was proposed to transmit heavy and bulky material. There was no experience for a guide.
The Clay-Lieb Patents.
—The Pneumatic Transit Company at this time turned to the Electro-Pneumatic Transit Company, of New Jersey, a national company that31 had been in existence since 1886, and which claimed to own valuable patents, for the ways and means to carry out its new enterprise. The patents were those of Henry Clay and Charles A. Lieb, and the rights to use them in the State of Pennsylvania were procured by the Pneumatic Transit Company, under a contract entered into between the two companies. The patents claimed to cover a practical working system by which a large number of stations could be connected to a system of main and branch tubes, with electrically-operated switches at the junctions of the branches with the main lines. Any person who gives the subject a little thought will at once see the advantages of such a system if it could be made to operate. Up to the present time only single- or double-line tubes have been used, without branches. In the European systems, frequently several stations are located along a line, but the carriers must stop at each station, be examined, and if they are destined for another station, they must be redespatched. The cash systems used in many of our large stores have independent tubes running from the central cashier’s desk to each station about the store. It is plain to be seen that, if several of these stations could be connected by branches to a main tube, a large amount of tubing would be saved—a most desirable result. The advantages of such a system would be still greater for long lines of tube laid under the pavements, extending to stations located in different parts of a large city. It was such a result that the patents of Clay and Lieb aimed to accomplish.
In order to demonstrate the practicability of the system, the Electro-Pneumatic Transit Company had constructed32 in the basement of the Mills Building, on Broad Street, New York, a short line of small brass tubing, about two or three inches in diameter, with one branch, thus connecting three stations together. The tube was very short, probably not more than two hundred feet in length. The air-pressure required was very slight, probably not more than an ounce or two, being supplied by a small blower run by an electric motor.
At the junction of the branch and the main tube was located a switch that could be moved across the main tube and so deflect the approaching carrier into the branch. This switch was moved by an electro-magnet, or solenoid, that could be excited by pressing a button at the station from which the carrier was sent. When the carrier passed into the branch tube it set the switch back into its normal position, so that a second carrier, following the first, would pass along the main tube, unless the switch was again moved by pressing the button at the sending station.
This tube in the Mills Building worked well, but it was of a size only suited to the transmission of cash in a store or other similar service. It could not be said, because this tube worked well, that a larger and longer tube with numerous branches would work equally well. In fact, there are several reasons why such a tube would not operate satisfactorily. The method of operating the switches was impracticable. Suppose the branch tube had been located two miles away from the sending station and that it would take a carrier four minutes to travel from the sending station to the junction of the branch tube. Again, suppose that we have just despatched a carrier destined for a station33 on the main line beyond the junction, and that we wish to despatch the second carrier to be switched off into the branch tube, we must wait at least four minutes, until the first carrier has passed the junction, before we can press the button and set the switch for the second carrier which may be on its way. How are we to know when the first carrier has passed the junction, and when the second will arrive there, in order that we may throw the switch at the proper time? Must we hold our watch and time each carrier? It is plain that this is not practical. I take this as an illustration of the impracticability of the Clay-Lieb System as constructed in the Mills Building when extended to practical dimensions. I will not describe the mechanism and details of the system, which are ingenious, but will say in passing that the automatic sluice-gates, which work very well in a three-inch tube with carriers weighing an ounce or two and air-pressures of only a few ounces per square inch, would be useless and could not be made to operate in a six-inch tube with carriers weighing from eight to twenty-five pounds and an air-pressure of from five to twenty-five pounds per square inch. For further information the reader is referred to the patents of Clay and Lieb.
Franchises and First Government Contract.
—In the spring of 1892 an ordinance was passed by Common and Select Councils, and signed by the Mayor of the City of Philadelphia, permitting the Pneumatic Transit Company to lay pneumatic tubes in the streets of that city. At the time this franchise was granted negotiations were in progress with the post-office department, in Washington, for the construction of a six-inch pneumatic34 tube, connecting the East Chestnut Street sub-post-office, at Third and Chestnut Streets, with the main post-office, at Ninth and Chestnut Streets, for the transmission of mail. This sub-post-office was selected because more mail passes through it daily than any other sub-office in the city, it being located near the centre of the banking district. Negotiations were delayed by various causes, so that the contract with the government was not signed until October, 1892.
Search for Tubes.
—It was at this time that the writer was first employed by the Pneumatic Transit Company, as engineer, to superintend the construction of this line. The company commenced at once to carry out its contract with the United States government, both the post-office department and the company being very desirous of having the work completed before winter. The time was very short for such an undertaking, but wrought-iron tubes had already been ordered of a well-known firm who manufacture pipe and tubing of all kinds. After waiting four or five weeks the first lot of tubes were finished, but upon inspection it was found that they were not sufficiently accurate and smooth on the interior to permit of their being used for the purpose intended. The next thing that suggested itself was seamless drawn brass tubes. While they would be very expensive, the process of manufacture makes them eminently suited for the purpose, but they could not be obtained in time. A city ordinance prohibits the opening of the streets of Philadelphia during the winter months except in extreme cases. Accurate tubes must be had, and had quickly. It then occurred to the writer that it might be possible to bore a sufficient quantity35 of ordinary cast-iron water-pipe and fit the ends accurately together to answer our purpose. Inquiry was made at nearly all the machine-shops in the city, to ascertain how many boring-machines could be put upon this work of boring nearly six thousand feet of six-inch pipe. It was found impossible to get the work done in time, if it was to be done in the usual manner of boring with a rigid bar. At last a man was found in Mr. A. Falkenau, engineer and machinist, who was prepared to contract for the construction of twelve special boring-machines and to bore all the tubing required. Suffice it to say, that the machines were built, and about six thousand feet of tubes were bored, between November 8 and December 31.
Method of Manufacturing Tubes.
—The process of boring was novel in some respects, and might be termed reaming rather than boring. Figs. 4 and 5 show the interior of the shop and the twelve machines. Fig. 6 is a drawing of one of the machines. A long flexible bar rotated the cutter-head, which was pulled through the tube, in distinction from being pushed. In order to give the feeding motion, a screw was attached to the cutter-head and extended through the tube in advance of it. The feed-screw was drawn forward by a nut attached to a hand-wheel located at the opposite end of the tube from which the boring began. Since it was not necessary that the tubes should be perfectly straight, a method of this kind was permissible, in which the cutters could be allowed to follow the cored axis of the tube. Air from a Sturtevant blower was forced through the tubes during the process of boring, for the double purpose of clearing the chips from37 the cutters and keeping them cool. After the tubes were reamed, each piece had to be placed in a lathe, have a counter-bore turned in the bottom of the bell, and have the other end squared off and turned for a short distance on the outside to fit the counter-bore of the next section.
Laying and Opening the Tubes for Traffic.
—The first tubes were laid about the middle of November, but December 1 came before the work was completed and special permission had to be obtained from the city to carry on the work after that date. All work was suspended during the holidays in order not to interfere with the holiday trade of the stores on Chestnut Street. Severe frosts prevailed at that season, so that when the work was begun again, after the holidays, bonfires had to be built in the streets to thaw out the ground in order to take up the paving-stones and dig the trench for the tubes. Several times the trench was filled with snow by unusually heavy storms. Notwithstanding all these delays and annoyances, the work was pushed forward, when a less determined company would have given it up, and was finally completed. A formal opening took place on February 17, 1893, when Hon. John Wanamaker, then Postmaster-General, sent through the tube the first carrier, containing a Bible wrapped in the American flag.
It was certainly a credit to the Pneumatic Transit Company and its managers that they were able to complete this first line of tubes so quickly and successfully under such trying circumstances. The tubes have been in successful operation from the opening until the present time, a period of nearly four years, and the repairs that38 have been made during that time have not required its stoppage for more than a few hours.
In the summer of 1895 the sub-post-office was removed from Chestnut Street to the basement of the Bourse (see Fig. 7). This required the abandonment of a few feet of tube on Chestnut Street and the laying of a slightly greater amount on Fourth Street, thus increasing the total length of the tubes a little. Wrought-iron tube, coated with some alloy, probably composed largely of tin or zinc, was used for this extension. The wrought-iron tube is not as good as the bored cast iron.
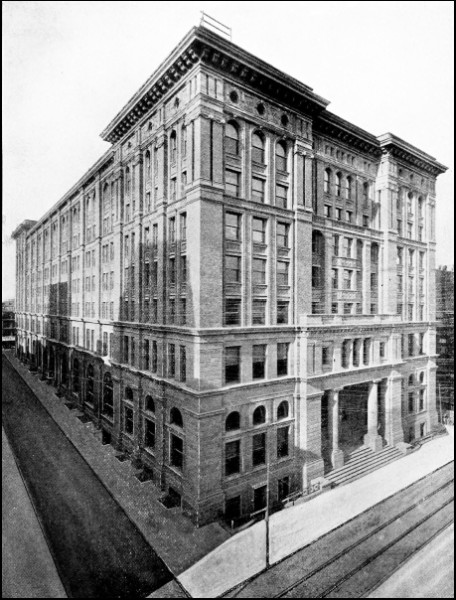
Fig. 7.
BOURSE BUILDING, PHILADELPHIA.
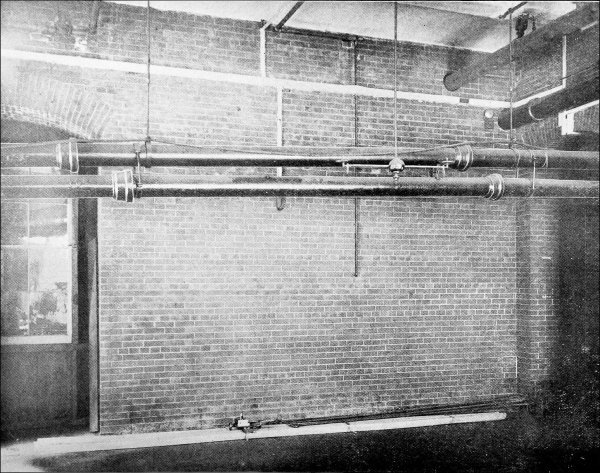
Fig. 8.
PNEUMATIC TUBES SUSPENDED IN THE BASEMENT OF THE MAIN POST-OFFICE.
Description of the Tubes, Method of Laying, etc.
—This Chestnut Street line consists of two tubes, one for despatching carriers from and the other to the main post-office. The distance between the two stations is two thousand nine hundred and seventy-four feet, requiring five thousand nine hundred and forty-eight feet of tube. The inside diameter of the tube is six and one-eighth inches, and it was made in sections each about eleven feet long, with “bells” cast upon one end, in order to join the sections with lead and oakum, calked in the usual manner of making joints in water- and gas-pipes, with this exception, that at the bottom of the bell a counter-bore was turned to receive the finished end of the next section. By thus machining the ends of each section of tube and having them fit accurately together, male and female, a practically continuous tube was formed with no shoulders upon the interior to obstruct the smooth passage of the carriers. Joints made in this way possess another great advantage over flanged and bolted joints, in that they are slightly yielding without39 leaking, and so allow for expansion and contraction due to changes of temperature. Each joint takes care of the expansion and contraction of its section, which is very slight, but if all were added together would amount to a very large movement. Another advantage of the “bell” joint is that it permits slight bends to be made in the line of tube without requiring special bent sections. Where short bends had to be made, at street corners, in entering buildings, and other similar places, brass tubes were used, bent to a radius of not less than six feet, or about twelve times the diameter of the tube. (One of the brass bends may be seen in Fig. 10.) The bends were made of seamless tubing, bent to the desired form and radius in a hydraulic machine. To prevent them from being flattened in the process of bending, they were filled with resin, which was afterwards melted out. Flanges were screwed and soldered to the ends of the bent brass tubes, and they were bolted to special flanged sections of the iron tube.
The tubes were laid in the trench and supported by having the ground firmly tamped about them. Usually one tube was laid above the other, with an iron bracket between, but frequently this arrangement had to be departed from in order to avoid obstructions, such as gas- and water-pipes, sewers, man-holes, etc. The depth of the tubes below the pavement varied from two to six feet, and in one place, in order to pass under a sewer, the extreme depth of thirteen feet was reached. At the street crossings it was frequently difficult to find sufficient space to lay the tubes. At the intersection of Fifth and Chestnut Streets a six-inch water-main had to be cut and a bend put40 in. A seven-strand electric cable, used for telephoning and signalling, was laid upon the top of one of the tubes, protected by a strip of “vulcanized wood,” grooved to fit over the cable. The cable and protecting strip of wood were fastened to the tube by wrought-iron straps and bolts.
The tubes enter the main post-office on the Chestnut Street side, through one of the windows, and are suspended from the ceiling along the corridor in the basement for a distance of nearly two hundred feet. Fig. 8 shows the tubes thus suspended. They terminate upon the ground floor about the centre of the building, and near the cancelling machines.
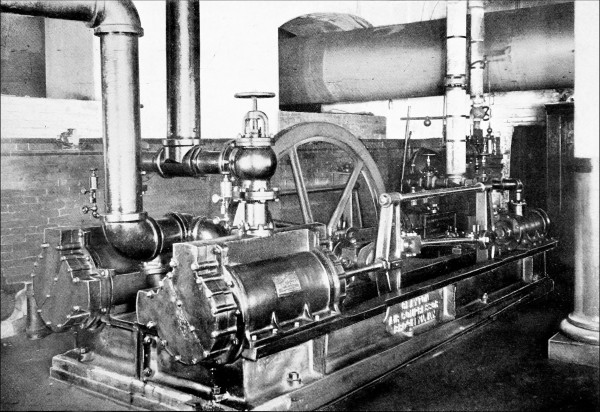
Fig. 9.
DUPLEX AIR-COMPRESSOR IN THE BASEMENT OF THE MAIN POST-OFFICE.
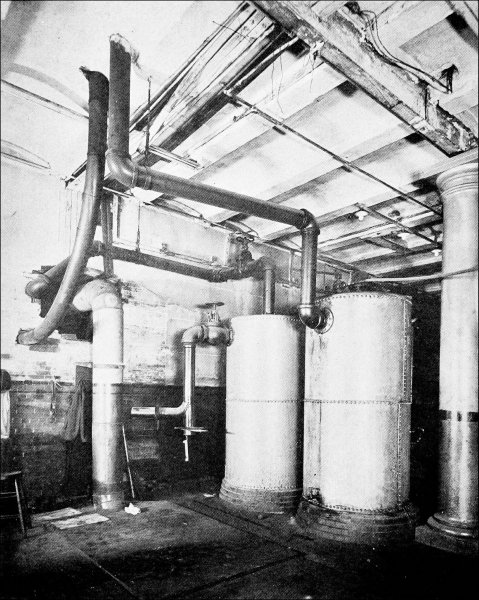
Fig. 10.
TANKS AND TUBE IN THE BASEMENT OF THE MAIN POST-OFFICE.
Air-Compressor—Method of Circulating the Air.
—The current of air that operates the tubes is supplied by a duplex air-compressor located in the basement of the main post-office. This machine is shown in Fig. 9, and requires no detailed description, as it does not differ materially from air-compressors used for other purposes. The stroke is twenty-four inches, the diameter of the steam-cylinders ten inches, and the air-cylinders eighteen inches. The air-cylinders are double acting, with poppet-valves, and have a closed suction. The speed of the machine varies slightly, being controlled by a pressure-regulator that maintains a practically constant pressure in the tank that feeds the tube. The engines develop a little over thirty horse-power under normal conditions. The pressure of the air as it leaves the compressor is usually six or seven pounds per square inch. Compressing the air heats it to about 156° F., but this is not sufficient to require water-jackets about the air-cylinders. From the compressor the air41 flows to a tank, shown on the right in Fig. 10, where any oil or dirt contained in the air is deposited. The principal purpose of the tank is, however, to form a cushion to reduce the pulsations in the air caused by the periodic discharge from the cylinders of the compressors, and make the current in the tube more steady. From this tank the air flows to the sending apparatus on the ground floor of the post-office and thence through the outgoing tube to the sub-post-office. At the sub-post-office, after flowing through the receiving and sending apparatus, it enters the return tube and flows back to the main office, passing through the receiving apparatus there and then to a tank in the basement,—the left tank in Fig. 10. The air-compressor draws its supply from this tank, so that the air is used over and over again. This return tank has an opening to the atmosphere, which allows air to enter and make up for any leakage or escape at the sending and receiving apparatus, thereby maintaining the atmospheric pressure in the discharge end of the tube and in the suction of the compressor. The tank serves to catch any moisture and dirt that come out of the tube. Fig. 11 is a diagram showing the direction and course of the air-current. It will be noticed that both the out-going and return tube are operated by pressure, in distinction from exhaust. The air is forced around the circuit by the air-compressor. There is no exhausting from the return tube. The pressure of the air when it enters the tube at the main post-office is, say, seven pounds per square inch; when it arrives at the sub-post-office the pressure is about three and three-quarters pounds, and when it gets back to the42 main office and enters the return tank, the pressure is zero or atmospheric. Thus it will be seen that the pressure becomes less and less as the air flows along the tube. This is not the pressure that moves the carriers, but the pressure of the air in the tube, a pressure that exists when there are no carriers in the tube. It is the pressure that would be indicated if you should drill a hole into the tube and attach a gauge.
Terminal Apparatus.
—When the construction of this line was begun, it was the intention of the Pneumatic Transit Company to use the apparatus of the Electro-Pneumatic Transit Company, at both stations, for sending and receiving carriers, and so-called working-drawings were obtained for this purpose. The sending apparatus was constructed according to the designs furnished, but, upon examination of the drawings of the receiving apparatus, it was so apparent that it would not work as intended that it was never constructed.
The writer was asked to design an automatic receiver43 to stop the carriers without shock upon their arrival at the stations, and to deliver them upon a table without appreciable escape of air,—something that would answer the requirements of the present plant.
The Sender.
—The sending apparatus is for the purpose of enabling the operator to place a carrier in the tube without allowing the air to escape. In other words, it is a means of despatching carriers. The apparatus for this purpose, already referred to, is simply a valve. A side view and section of it are shown in Fig. 12. Fig. 15 is a view of the apparatus in the main post-office. The sending apparatus is seen on the left. Fig. 13 is a view of the sub-post-office apparatus, and here a man may be seen in the act of despatching a carrier. Referring to44 the section, Fig. 12, it will be seen that the sending apparatus consists of a short section of tube supported on trunnions and enclosed in a circular box. Normally this short section of tube stands in line with the main tube, and the air-current passes directly through it. It is shown in this position in the figure. When a carrier is to be despatched, this short section of tube is rotated by a handle until one end comes into coincidence with an opening in the side of the box. In this position the air flows through the box around the movable tube. A carrier can then be placed in the short section of tube and be rotated by the handle into line with the main tube. The carrier will then be carried along with the current of air. A circular plate covers the opening in the box where the carrier is inserted when the sending apparatus is closed.
At the sub-post-office this sending apparatus is placed in a horizontal position, but its operation is the same.
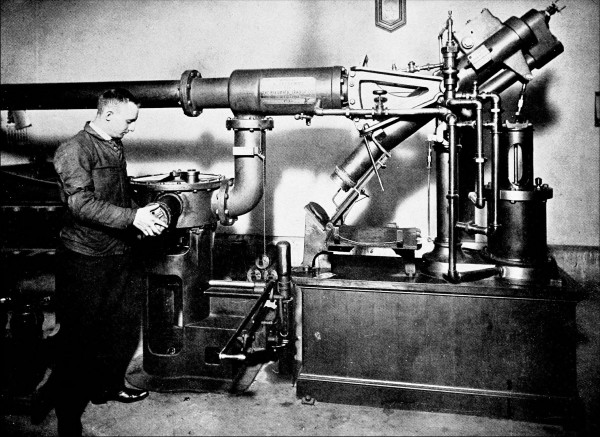
Fig. 13.
RECEIVING AND SENDING APPARATUS IN THE SUB-POST-OFFICE.
Sub-Post-Office Receiver.
—We have already explained that the air-pressure in the tube at the sub-post-office is about three and three-quarters pounds per square inch. With such a pressure we cannot open the tube to allow the carriers to come out. They must be received in a chamber that can be closed to the tube after the arrival of a carrier and then opened to the atmosphere. Furthermore, this chamber must act as an air-cushion to check the momentum of the carriers. Fig. 13 shows the sub-post-office apparatus when a carrier is being delivered from the receiving apparatus, or, as we will name it for convenience, the receiver. Fig. 14 is a drawing of the same apparatus, partly in section, that shows more clearly its method of46 operation. This drawing shows the sending apparatus in a different position from Fig. 13, but that is immaterial. The receiver consists of a movable section of tube, about twice the length of a carrier, closed at one end, supported upon trunnions, and normally in a position to form a continuation of the main tube from which the carriers are received. When a carrier arrives it runs directly into the receiver, which being closed at the end forms an air-cushion that stops the carrier without shock or injury. Just before reaching the receiving chamber the current of air passes out through slots in the walls of the tube into a jacket that conducts it to the sending apparatus, as shown in Fig. 14. At the closed end of the receiving chamber, or air-cushion, is a relief valve, normally held closed by a spring. As the carrier compresses the air in front of it, this valve opens and allows some of the air to escape, which prevents the carrier from rebounding into the tube. Under the outer end of the receiving chamber is a vertical cylinder, E, Fig. 14, supported upon the base-plate containing a piston. The piston of this cylinder is connected by a piston- and connecting-rod to the receiving chamber. When air is admitted to the cylinder under the piston, the latter rises and tilts the receiving chamber to an angle of about forty degrees, which allows the carrier to slide out. The receiving chamber carries a circular plate, C, that covers the end of the main tube when it is tilted. A small piston slide-valve, F, located near the trunnion of the receiving chamber, controls the admission and discharge of air to and from the cylinder E, upon the arrival of a carrier. When a carrier arrives and47 compresses the air in the air-cushion or receiving chamber, a small portion of this compressed air is forced through pipe G, to a small cylinder containing a piston and located just above the piston slide-valve F. The increased pressure acting on the piston moves it downward, and it in turn moves the slide-valve F. Thus it will be seen that the stopping of the carrier causes the receiving chamber to be tilted and the carrier slides out on to an inclined platform, K. This platform is hinged at one end, and supported at the angle seen in the figure by a counterweight. When a carrier rests upon it, the weight of the carrier is sufficient to bear it down into a horizontal position; in this position the carrier rolls off on to a table or shelf. The platform, K, is connected by rods, bell-cranks, etc., to the piston slide-valve, so that when it swings downward by the weight of a carrier, the slide-valve is moved upward into its normal position, and this causes the receiving chamber to tilt back into a horizontal position ready to receive the next carrier. The time that elapses from the arrival of a carrier until the receiving chamber has returned to its horizontal position is not more than three or four seconds. Nothing could operate in a more satisfactory manner.
Main Post-Office Receiver.
—At the main post-office we have a receiver of a different type. It will be remembered that the pressure in the return tube at the main post-office is nearly down to zero or atmospheric, so that we can open the tube to allow the carriers to pass out without noise or an annoying blast of air. Figs. 15 and 16 show the main-office apparatus, and Fig. 17 is a drawing of the same. Here the receiver consists of a section of48 tube closed by a sluice-gate, located at B, Fig. 17. The air-current passes out through slots in the tube into a branch pipe leading to the return tank in the basement. These slots are located about four feet back of the sluice-gate, so that the portion of the tube between the slots and the sluice-gate forms an air-cushion to check the momentum of the carriers. The sluice-gate is raised and lowered by a piston moving in a cylinder located just above the gate. The movement of this piston is controlled by a piston slide-valve in a manner similar to the apparatus at the sub-post-office. Air for operating the piston is conveyed through the pipe D, Fig. 17, from the pipe leading from the air-compressor to the sending apparatus. This air is at about seven pounds pressure per square inch.
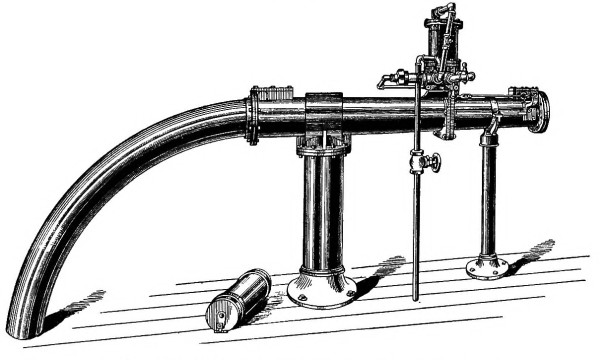
Fig. 16.
RECEIVING APPARATUS AT THE MAIN POST-OFFICE.
When a carrier arrives, after passing the slots that allow the air-current to flow into the branch pipe, it compresses50 the air in front of it against the gate. This compression checks its momentum, and it comes gradually to rest. The air compressed between the carrier and the sluice-gate operates to move the piston slide-valve, thereby admitting air to the gate cylinder under the piston, which rises, carrying with it the sluice-gate. The tube is now open to the atmosphere, and there is just sufficient pressure in the tube to push the carrier out on to a table arranged to receive it. As the carrier passes out of the tube it lifts a finger out of its path. This finger is located at E, Fig. 17, and when it is lifted by the passing carrier it moves the piston slide-valve, and the sluice-gate is closed. A valve is located in the branch-pipe that conducts the air to the return tank in the basement. If the pressure in the tube is not sufficient to push the carrier out on to the table, this valve is partially closed, thereby increasing the pressure to a desired amount.

Fig. 18.
CARRIER.
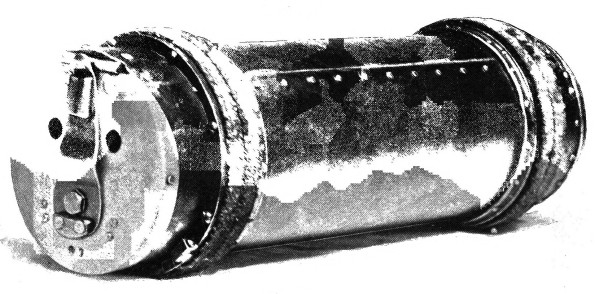
Fig. 19.
CARRIER.
The Carrier.
—We have frequently spoken of the carrier, which contains the mail and other parcels that are transported from one office to the other. In Fig. 13, showing the sub-post-office apparatus, we see one of these carriers being despatched by the attendant and another being delivered from the tube. In Fig. 15 several carriers may be seen standing on the floor. Fig. 18 shows a carrier with the lid open, ready to receive a charge of mail, and Fig. 19 shows the same closed, ready for despatching. The construction of the carrier is shown by the drawing, Fig. 20. The body of the carrier is steel, about one-thirty-second of an inch in thickness. It is made from a flat sheet, bent into a cylinder, riveted, and soldered. The51 length outside is eighteen inches, and the inside diameter is five and one-quarter inches. The front end is made of a convex disk of steel, stamped in the desired form, and secured to the body of the carrier by rivets, with the convex side inward. It is necessary to have a buffer upon the front end of the carrier to protect it from blows that it might receive, and this buffer is made by filling the concave side of the front head with felt, held in place by a disk of leather and a central bolt. The leather disk is made of two pieces, riveted together, with a steel washer between. The steel washer is attached to the head of the bolt. The carrier is supported in the tube on two bearing-rings, located on the body of the carrier a short distance from each end. The location of these rings is so chosen that it permits a carrier of maximum length to pass through a bend in the tube of minimum radius without becoming wedged. This is a very important feature in the construction of carriers, but does not appear to have been utilized in other systems.
The bearing-rings are made of fibrous woven material, especially prepared, and held in place by being clamped between two metal rings, one of which is riveted to the52 body of the carrier. Of course these rings wear out and have to be replaced occasionally, but their usual life is about one thousand miles. The rear end of the carrier is closed by a hinged lid and secured by a special lock. The lock consists of three radial bolts that pass through the body of the carrier and the rim of the lid. These bolts are thrown by three cams, attached to a short shaft that passes through the lid and has a handle or lever attached to it upon the outside of the lid. This cam-shaft is located out of the geometrical centre of the lid in such a position that when the lever or handle is swung around in the unlocked position, it projects beyond the periphery of the lid, and in this position the carrier will not enter the tube. When the lid is closed and locked, the lever lies across the lid in the position shown in Fig. 19, and when the carrier is in the tube it cannot become unlocked, for the lever cannot swing around without coming in contact with the wall of the tube. This insures against the possibility of the carriers opening during transit through the tube. The empty carriers weigh about nine pounds, and when filled with mail, from twelve to fifteen pounds. They have a capacity for two hundred ordinary letters, packed in the usual manner.
Operation of the Tubes.
—The tubes are kept in constant operation during the day, and six days of the week. The air-compressor is started at nine o’clock in the morning and runs until seven in the evening, except during the noon hour, the air flowing in a constant steady current through the tubes. When a carrier is placed in the tube it is carried along in the current without appreciably affecting the load on the compressor. Carriers may be despatched53 at six-second intervals, and when they are despatched thus frequently at each office, there will be eighteen carriers in the tube at the same time. If ten carriers per minute are despatched from each office, and each carrier contains two hundred letters, the tube has a carrying capacity of two hundred and forty thousand letters per hour, which is far beyond the requirements of this office. About five hundred carriers a day are despatched from each office. This varies considerably on different days and at different seasons of the year. Experience has taught that a certain period of time should elapse between the despatching of carriers, in order that they may not come in contact with each other, and that the receivers may have time to act. With the present plant this period is made about six seconds. In order to make it impossible for carriers to be despatched more frequently than this, time-locks are attached to the sending apparatus. One of these locks may be seen in Fig. 13, connected to the handle of the sending apparatus. It is so arranged that when a carrier is despatched a weight is raised and allowed to fall, carrying with it a piston in a cylinder filled with oil. While the weight is rising and falling the sending apparatus is locked, but becomes unlocked when the weight is all the way down. A by-pass in the cylinder permits the oil to flow from one side of the piston to the other, and the size of this by-pass can be regulated, thus determining the time that the weight shall take in descending. This makes a simple and effective time-lock that does not get out of order.
The time required for a carrier to travel from the main to the sub-post-office is sixty seconds, and from the sub- to54 the main post-office, fifty-five seconds. This difference of time in going and returning is due to the expansion of the air in the tube, as will be explained more fully in another place. The distance between the offices being two thousand nine hundred and seventy-four feet, gives an average speed of about fifty-two feet per second, or 35.27 miles per hour. Of course the speed can be increased by increasing the air-pressure, but this speed is found in practice to be ample for all requirements. In order to give some idea of the energy possessed by one of these carriers travelling at this speed, it may be said that if the end of the tube were left open and turned upward, an emerging carrier would rise about forty feet into the air. It is easy to imagine how apparatus, depending for its operation upon impact with a moving carrier, would be soon destroyed, as well as the carriers themselves. This is why receiving apparatus used with small tubes and light carriers cannot be applied to large tubes with heavy carriers.
No serious trouble has ever been experienced from carriers getting wedged in any part of these tubes.
Benefits of the System.
—The advantages to the post-office department by the adoption of this system have been numerous, and the post-office officials who are familiar with the operation of the tubes frequently speak in high terms of their usefulness. Formerly the mail was transported from one office to the other by a wagon making a trip every half-hour. Considerable time has been saved by the greater speed of transit, but even more time is gained by keeping the mail moving instead of allowing it to accumulate and then despatching it in bulk. With the55 pneumatic system a letter posted in the sub-post-office will reach its destination just as quickly as if posted at the main office, and sometimes more quickly. Let us take an example, first, with the old wagon service. Suppose that you drop a letter in the sub-post-office; it lies there, say, fifteen minutes waiting for the departure of the next wagon; it is put into a pouch with hundreds of other letters, and ten minutes are consumed in transporting it to the main office. When it arrives there the pouch is thrown on the floor at the entrance of the building; in a few minutes, more or less, a clerk takes the pouch, throws it on a truck and wheels it around to the cancelling machines, where it may lie for five or ten minutes more before being opened, and then perhaps five minutes will elapse before your letter reaches the cancelling machine. It would not be unusual for three-quarters of an hour to elapse from the time you dropped your letter in the office until it was cancelled. Now with the pneumatic tube service forty minutes of this time will be saved; for immediately after you drop your letter in the office it will be despatched through the tube and delivered on the table in front of the cancelling machines. Soon after the tubes were installed the postmaster’s attention was called to an instance where letters from the sub-office were sent through the tube and were despatched to New York City one train earlier than they could have been had the old wagon service been in use. People frequently post letters requesting that they be sent through the tube; of course they would be sent in that way if the request was not made, but it shows that the public recognize the better56 service. Formerly mail was collected from the street boxes in the banking section of the city and the collectors carried it to the main office. After the tubes were installed this mail was carried to the sub-post-office to be sent through the tube, and the time formerly occupied in walking to the main office was then utilized in having the men face up the letters ready for the cancelling machines,—a double saving in time besides making their labor much lighter and enabling them to do more useful work.
Since the sub-office has been established in the Bourse, it has been made a distributing as well as receiving office. At least two more deliveries of mail are made each day in the Bourse building than in any other office building in the city.
All letters mailed in the sub-office with a special delivery stamp are despatched through the tube immediately.
It is now nearly four years since the system was put into operation. During that time more than thirty-five million letters have been transported, and all the repairs to the system have not required it to be stopped for more than a few hours. During the first year the Pneumatic Transit Company operated the tubes at their own expense, agreeing at the end of that time to take them out if the government so requested. Since the first year the government has paid the running expenses.
Such is the history of the first United States pneumatic postal system. Such is the history of the first pneumatic tubes of sufficient size to carry all the first class and most of the lower classes of mail, in this or any other country, so far as the writer knows.
CHAPTER III.
THE SYSTEM AND APPARATUS OF THE BATCHELLER PNEUMATIC TUBE COMPANY.
General Arrangement and Adaptability of the System.
—The experience gained in the construction and operation of the Philadelphia post-office tubes has naturally suggested improvements that can be made in future construction, and, furthermore, it has taught us what the requirements will be of an extensive system of tubes laid in the streets of our cities, both for the transmission of mail and for a general commercial business. Since the Philadelphia post-office tube was completed, we have been busily engaged in working out all the details of a system of many stations so connected together that carriers can be despatched in the most direct manner possible from any station to any other. It is the purpose of the present chapter to describe this system.
While the Pneumatic Transit Company has ample field in the State of Pennsylvania to carry out the work which it has mapped out, a field broad enough to yield a good profit for the capital invested, there is no reason why the system should be limited to one State. So, in order to obtain a broader charter, covering all places where pneumatic service may be needed, a new corporation was formed and styled the Batcheller Pneumatic Tube Company.
It is impossible to lay down a rigid system equally well58 adapted to all places and purposes. We must accommodate ourselves somewhat to circumstances. For example, the post-office department may require one size of tube, arranged to operate in a particular way, while the requirements of a parcel delivery business would be utterly different. The geographical location of the stations will have much to do with the general arrangement; also the condition of the streets. Some of the streets of our large cities are so filled with water- and gas-pipes, electrical conduits, sewers, steam-pipes, etc., etc., that it is almost impossible to find space for pneumatic tubes, especially of large diameter. Railway or water facilities have much to do with the location of a central pumping station, on account of the coal supply. All of these and many other things have to be taken into consideration in planning a system for any locality.
We have an example of a peculiar location and conditions in a proposed line of tubes over the New York and Brooklyn bridge connecting the main post-offices of those cities. This would be in many respects a unique plant. Two air-compressors would be used, one at each office.
In order to give a general idea how a large number of stations can be connected into one system, the diagram Fig. 21 has been drawn.
We have already referred to the attempts of Clay and Lieb to devise means whereby several stations could be located along a main line and carriers be sent from any station to any other through the main line. Their method was to use branch tubes leading off from the main line with switches at the junctions. They deflected the air-current59 into the branch by placing an automatic closed valve in the main line just beyond the junction, returning the air from the branch to the main line just beyond the valve. The carriers were to open and close this valve automatically as they passed.
The branch and switch system has many attractions for the inventor, and upon first thought it would seem the most feasible solution of the problem. It has been the dream of more than one inventor, as the records of the patent-office show, but no one has succeeded in working it out. The current of air cannot be divided; carriers passing from the branch into the main line must not collide with other carriers running in the main line; a certain minimum distance must always be maintained between the carriers in the same tube; when a carrier is despatched it must go directly to the station for which it is intended without further attention from the sender and it must not interfere with other carriers; expense of manufacture prohibits the use of any but round smooth tubes up to eight inches in diameter, hence projections cannot be placed upon the carrier to give it an individuality and cause it to operate a switch at any particular point along the line; the carrier is free to rotate in a round tube about its longitudinal axis, therefore, its individuality must be indicated by some symmetrical marking about this axis, if it is to be automatic in its operation; the speed of the carrier is so high that electrical contacts placed in distinctive positions on the carriers cannot be used while it is in motion, for mechanism having inertia could not be moved during the short time that the electric circuit would be closed; only60 the simplest attachments can be made to the carrier, for constructional reasons and because of the rough usage that they receive. These and numerous other reasons make the problem most difficult. We have not attempted to solve it by the use of branch tubes and electrically operated switches, but have adopted the simpler and equally effective method of carrying the main line through each of the stations that it unites. In our system each carrier has an individuality determining the station at which it will be discharged from the tube. By a simple attachment to, the front end of the carrier, consisting of a circular metal disk, the sender so marks the carrier that it will pass all stations until it arrives at the station for which it was destined and will there pass out of the tube. In addition to this a method has been devised whereby carriers can be inserted into the tube without the possibility of collision with carriers already running in the tube.
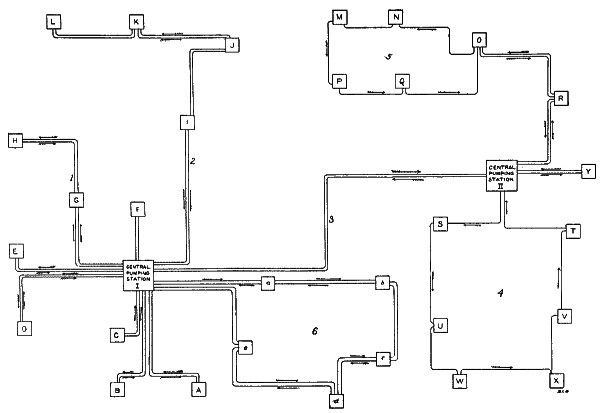
Fig. 21.
A DIAGRAM SHOWING VARIOUS METHODS OF CONNECTING THE STATIONS OF A LARGE
SYSTEM WITH PNEUMATIC TUBES.
Larger image (94 kB)
Referring now to the diagram, Fig. 21, we have here an imaginary system which we will suppose to be located in some large city. The two large squares I and II indicate central pumping stations, and the small squares A, B, C, D, etc., indicate receiving and sending stations. Some of the stations, such as A, B, C, D, E, F, and Y, which do a large amount of business and may be supposed to be large retail stores, are connected directly with the central station by double tubes, one for sending and the other for receiving carriers. Two smaller stores, such as G and H, may be located on the same line. At I, J, K, and L we have four stations, all connected by the same double line of tubes. These stations we will imagine to be located in the residence62 section of the city. Carriers containing parcels of merchandise or other matter destined for private residences would be sent from the stores A, B, C, etc., to the central station I, where they would be transferred to the line 2 and be adjusted to stop at the station nearest the residence to which the parcels were addressed. From this station the parcels would be delivered by messengers to the residences. If a carrier is to be sent from the central station I to station K, it will be so adjusted before it is put into the tube that it will pass stations I and J, but be discharged automatically from the tube when it arrives at station K. In a similar manner carriers can be despatched from station L to station I or from station J to station L. In passing through the central station the carriers are manually transferred from one line to another.
In another part of the city we may have another central pumping station, II; and the two central stations may be connected by a double trunk line, 3. Again, we have lines radiating from this central station, as shown by station Y. There will be some localities where it will be an advantage to arrange the stations upon a loop, as shown in circuit 4, where stations S, T, U, V, W, and X are connected together in this way. Or we can combine the two arrangements of loop and direct line, as shown in circuit 5. Stations O and R are on the double line, but from O a loop is formed including stations N, M, P, and Q. Here it is supposed that the stations O and R do a much larger business with the central station II than the stations N, M, P, and Q, this being the principal reason for placing them on the double line. All carriers must be returned to the station from63 which they were sent, or others to replace them, otherwise there will be an accumulation of carriers at some of the stations. It is like a railway: there must be as many trains despatched in one direction as the other, each day. Station O can receive a carrier from the central station and return it directly, but when station N receives one it must be returned via M, P, Q, O, and R, a much longer route than that by which it was received. This disadvantage is compensated, when stations N, M, P, and Q do only a small amount of business, by the less cost of laying a single line. If a carrier is to be sent from M to N, it must go via P, Q, and O, being manually transferred at O from the “down” to the “up” line. P can send directly to Q, but Q must send to P via O, N, and M. R can send directly to O and O to R. Similarly in circuit 4 the carriers must all travel around the loop in the same direction, shown by the arrows. Station S can receive carriers directly from the central station, but they must return via U, W, X, V, and T.
Again, we may have a double-loop line, as indicated in the diagram by circuit 6. Here five stations, a, b, c, d, and e, are connected by a loop consisting of two lines of tube, in which the air circulates in one direction in one line and in the opposite direction in the other. Here b can send directly to c, c directly to b, and e to b via d and c, or via central and a. This is an arrangement that would be used where there is a large amount of business between the stations on the loop. As stated before, the best arrangement for any particular locality depends entirely upon circumstances.
Size of Tubes.
—The pneumatic-tube system that we are describing is not limited to any particular size of tube. The size is usually determined by the number and size of packages to be transported. A small tube, two or three inches in diameter, is best suited for telegrams and messages; mail, parcels, etc., require a six- or eight-inch tube, while mail pouches and bulky material, a thirty-six inch or possibly larger tube. We divide tubes into three classes, according to their size, naming them small, large, and very large tubes. By small tubes we mean those not larger than three or possibly four inches in diameter. Large tubes are those having a diameter more than four inches and not more than eight inches. Very large tubes include all that are more than eight inches. This classification is for convenience, but it has a deeper significance. For example, in the transportation of mail, it must either be handled in bulk, that is, in pouches, or in broken-bulk, that is, loose or tied up in small packages. There are many advantages in transporting it in broken-bulk, in fact, there are very few places where it could be handled in any other way. For this service six- or eight-inch tubes—not larger—are best suited. The carriers are light enough to be easily handled; they are not so large in capacity as to make it necessary to wait for an accumulation of mail to fill them; they can be delivered from the tube on to tables at any point in the building where the mail is wanted, for cancelling, distribution, or pouching, thus rendering a very rapid service; the mail is kept moving in an almost constant stream, keeping the postal employees more uniformly employed; special carriers can be despatched with “special65 delivery” letters. In other words, the most rapid service can be rendered by this size of tube.
If a larger than eight-inch tube is to be used for mail service, it should be not less than thirty-six inches. Carriers larger than eight inches cannot be handled: they are too heavy. They are also too heavy to slide through the tube, hence, must be mounted upon wheels. It is not practical to make a carrier on wheels less than eighteen or twenty-four inches, and the carrier must be at least twenty-four inches to contain a large mail-pouch. Now, if we are going to despatch mail-pouches through a pneumatic tube we must send more than one in a carrier, otherwise the service will be too slow. Such large carriers could not be despatched oftener than once or at most twice in a minute. Suppose we were to transport the mail from a railway station to a main post-office. A train arrives with, say, sixty pouches. If only one pouch could be put into a carrier and the carriers could be despatched at half-minute intervals, it would take thirty minutes to despatch all the pouches. Now, suppose we make the tube thirty-six inches. The carriers will be eight feet long and will contain from twelve to fifteen pouches. Five carriers would contain the entire train-load of mail, and they could be despatched in four or five minutes.
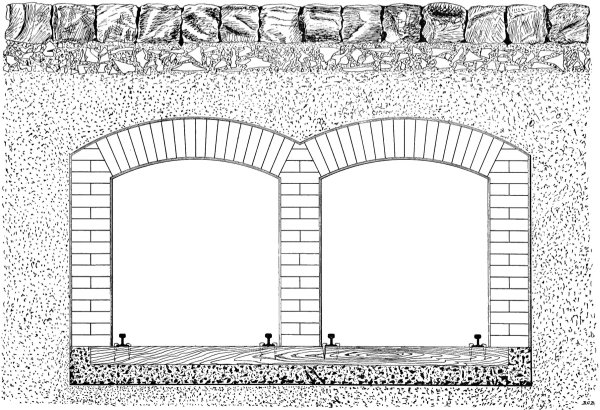
Fig. 22.
CROSS-SECTION OF A 36-INCH TUBE.
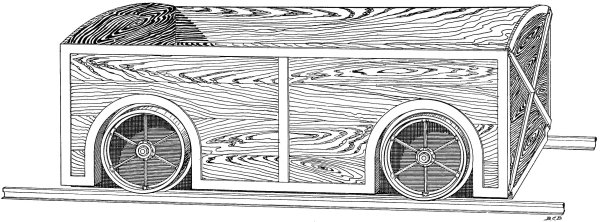
Fig. 23.
CARRIER FOR A 36-INCH TUBE.
System of Very Large Tubes.
—The cross-section of a thirty-six-inch tube is shown in Fig. 22. It is built flat on the bottom and sides, with an arched top. The floor is of concrete containing creosoted ties; the side walls and top are of brick, plastered with cement upon the interior. The two tubes may be built one above the other68 or side by side, depending upon the condition of the streets, but one common separating wall will serve for both. The carriers, one of which is shown in Fig. 23, run on two rails laid close to the sides of the tube. At curves a guard-rail is placed upon the side wall, making it impossible for a carrier to leave the track. The carriers are made of hard wood with an iron frame, and are as light as consistent with the service required of them. They are open on top. Their outside dimensions are thirty-four inches by thirty-four inches by eight feet. The sending and receiving apparatus for these very large tubes have to be specially designed for each particular station, so no attempt will be made here to describe them. The air-pressure required depends upon the length of the line. If it were not more than six or eight ounces a fan would be used to maintain the air-current, but for pressures above this, up to a pound or two per square inch, some form of positive blower would be used.
At the stations considerable floor space or “yard room” would be required for side tracks, switches, etc. Usually the basement of a building would have to be utilized for the termination of such a tube. There are but few places in our large cities where the streets are so free from pipes, sewers, conduits, etc., that it would be practicable to build a thirty-six-inch pneumatic tube. When the service can be rendered by an eight-inch tube, the cost of installation favors its adoption. Steep grades cannot be ascended by these very large tubes, while the eight-inch tubes can be placed vertically. We do not say that there is no use for eighteen- and twenty-four-inch tubes, but the69 demand for them would be in special cases and we will not discuss them here. For ordinary mail and parcel service we recommend the use of six- and eight-inch tubes. An eight-inch carrier is twenty-four inches long, about seven inches inside diameter, and will contain five hundred ordinary letters. It weighs about thirteen pounds empty, and one can be despatched every six to ten seconds. We estimate that eighty per cent. of all the parcels delivered from a large retail department store could be wrapped up to go into these carriers. The minimum radius of curvature of an eight-inch tube is eight feet.
General Arrangement of Apparatus in the Stations. Two-Station, Two-Compressor Line.
—We will now proceed to a description of our system in detail. Figs. 24, 25, and 26 are diagrams showing how the tubes, air-compressor, tanks, sending and receiving apparatus are connected together at the stations. These diagrams are drawn to represent an eight-inch tube, but essentially the same arrangement would be used for smaller tubes.
Fig. 24 represents a line of two stations with an air-compressor at each station. Such an arrangement is proposed for the line of postal tubes over the New York and Brooklyn bridge, or for any two stations located a very long distance apart, say six or eight miles.
Referring to the diagram, we have at station A an air-compressor, c, which draws its supply of air from the tank e, and delivers it, compressed to the necessary pressure, into the tank d. From the tank d the air flows to the sending apparatus, a, and thence through the tube f to the station B. Upon arrival at B it flows through the receiving71 apparatus m, and then by the pipe l to the tank j. A second air-compressor, o, is located at station B, and it draws its supply of air from the tank j. The tank j has an opening to the atmosphere, i, through which air can enter when the air-compressor draws more than is supplied from the pipe l. The opening i in the tank j serves as an escape for air when the air-compressor at station A is started before that at station B. Stations A and B are similar in their arrangements. At B the air-compressor o delivers its compressed air to the tank k, from which it flows to the sending apparatus n, and thence through the tube g back to station A. Upon its arrival at A it passes through the receiving apparatus and enters the tank e, which is open to the atmosphere at h. The tanks d and k serve as separators to remove from the air any dirt and oil coming from the compressors, and they form a cushion, deadening, to some extent, the pulsations of the compressors and making the current of air in the tubes more steady and uniform. The tanks e and j form traps to catch any moisture, oil, or dirt coming out of the tubes.
Carriers are placed in the tubes and despatched by means of the sending apparatus a and n. They are received from the tubes and delivered on to tables by means of the receiving apparatus b and m. It will be seen that the arrangement is such that the air flows through one tube and returns through the other, the same air being used over and over again. Any air that escapes at the sending and receiving apparatus is replaced by an equal amount entering the tanks e and j from the atmosphere. By thus keeping72 the same air circulating in the tubes we prevent an accumulation of moisture in the tubes.
The air is at its maximum pressure in the tanks d and k. The pressure falls gradually as it flows along the tubes and is down to atmospheric when it enters the tanks e and j. The pressure at the receivers, b and m, is just sufficient to push the carriers out on to the tables. The construction of the sending and receiving apparatus will be described in another place.
Two-Station, One-Compressor Line.
—Fig. 25 is a diagram showing two stations, A and B, connected by a double line of tubes, both operated by one air-compressor located at station A. This is the arrangement used in the Philadelphia post-office line, and is the arrangement that will ordinarily be used for all two-station lines except where unusual conditions require something different. Station A is arranged precisely like station A in Fig. 24, so it need not be described again. The air flows from the sending apparatus a through the tube f to the receiving apparatus p at station B. From the receiver p it flows through the pipe l to the sending apparatus n and thence through the tube g back to station A. The receiver p at station B is what we will call a closed receiver,—i.e., it delivers the carrier from the tube on to the table without opening the tube to the atmosphere. The use of this form of receiver is made necessary by the fact that the air-pressure in the tube at this station is considerably above atmospheric. The air-pressure is at a maximum in the tank d. It falls gradually along the tube f, and when the air arrives at the receiver p, at station B, the pressure has74 fallen nearly to one-half its maximum amount in the tank d. On its return journey through the tube g the pressure continues falling until it reaches the atmospheric pressure when the air enters the tank e at station A.
The entire line of tube, going and returning, is operated by air at a pressure above the atmospheric. There is no exhausting in the return tube. It is distinctly a pressure system.
Three- to Eight-Station Line.
—Thus far we have described only two-station lines. In Fig. 26 we have a diagram of three stations connected together by a double line of tubes, and the arrangement would be similar if it were extended to four, five, six, seven, or eight stations. The stations are called A, B, and H. Station A is arranged exactly the same as stations A in Figs. 24 and 25, therefore, needs no description. Station B, being an intermediate station, is quite differently arranged from any of the preceding. From station A the air flows through the tube f to station B, where it enters the automatic receiving and transferring apparatus, s. From this it flows through the tube f′ to the sending apparatus r, and thence through the tube f″ to the next station, which may be another intermediate station, C, or the terminal station H. Station H is arranged like station B, Fig. 25. The air from the tube f″ enters the receiver p, and is then returned, through the pipe l, to the sending apparatus n. From the sending apparatus n it continues on its return journey through the tube g to the intermediate station B, where it enters the receiver and transfer apparatus t, then passes 76to the sending apparatus q, and through the tube g″ back to the receiver b at station A. Thus we have followed the air-current out through one tube and back through the other. The current is kept circulating by the compressor located at station A. The pressure is at a maximum in the tank d, and falls gradually as the air flows along the tube until it returns to the tank e, when the pressure has fallen to atmospheric. A carrier is despatched from station A, and after passing through the tube f arrives at station B, where it stops momentarily in the automatic receiver and transfer apparatus s. If the carrier is intended for station B, and was properly adjusted when it was despatched at A, it will be discharged from the apparatus s on to the table u. But if it were intended for some other station and were so adjusted, after the delay of two or three seconds in the apparatus s, it will be automatically transferred to the tube f′, pass through the sending apparatus r, and go on its journey through tube f′ to the next station. If it is not discharged from the tube at any of the intermediate stations, it will finally arrive at the terminal station H and there stop. Just how the carriers are adjusted and the details of the receiving and transfer apparatus will be described hereafter. Carriers arrive at station B from H, or other stations on the line, through tube g, in the apparatus t, which either discharges them on to the table u or sends them on through the tube g′ and g″ to station A. Carriers are despatched from station B to station A by means of the sending apparatus q, and from station B to other stations along the line, C, D, E, F, G, and H, by means of the sending apparatus r. Thus, from B carriers can be sent and received in either direction. In order to77 prevent the possibility of a collision of carriers by attempting to despatch one at station B at the instant another is passing through the sending apparatus, an automatic lock is attached to each sending apparatus. Just outside the station B, say three hundred feet on each side, are located manholes, and in these manholes boxes are attached to the tube containing an electric circuit-closing apparatus, so arranged that when a carrier passes it will close an electric circuit leading to the sending apparatus in the station. These manholes and circuit-closers are shown and located on the diagram at v and w. Wires x and y lead from them to the sending apparatus r and q. When a carrier from station A passes the box v, it closes the electric circuit x, which sets a time-lock on the sending apparatus r, holding this apparatus locked, so that it is impossible to despatch a carrier for, say, twelve seconds, a sufficient time for the carrier coming from the station A to pass station B and get three hundred feet beyond it. After the twelve seconds have elapsed the sending apparatus is unlocked and a carrier can then be despatched. In a similar manner a carrier coming from station H, in passing the box w, closes the electric circuit y and locks the sending apparatus q for a sufficient length of time to let the carrier pass the station. This resembles, in some respects, the “block system” as used on railroads. A “block” about six hundred feet in length, depending upon the speed of the carriers, is made at each intermediate station with the station in the centre of the block. Whenever a carrier enters this “block” the sending apparatus at the station is locked, and a carrier cannot be inserted into the tube to collide78 with the one which is passing. It will be noted that a carrier in passing out of the “block” does not unlock the sending apparatus; this is done automatically at a definite time after the carrier entered the block. The unlocking is entirely independent of the carrier after it has entered the block, and the reason it is so arranged is this: suppose that a second carrier enters the “block” before the first one leaves it; if the first carrier unlocked the apparatus when it left the “block,” then it would be unlocked with the second carrier in the block and a collision might occur, but by arranging it as we have done, if a second carrier enters the “block” before the first has passed out, the sending apparatus remains locked for a period of time beginning with the arrival of the first carrier in the “block” and ending, say, twelve seconds after the arrival of the last carrier, which is sufficient time for the last carrier to pass out of the block. Of course, if a carrier becomes wedged in the tube a collision may occur, but this very seldom if ever happens. The details of the locking apparatus will be described in another place.
If stations A, B, ... and H were arranged on a loop, as shown in circuit 6, Fig. 21, then station H, Fig. 26, would be at the central, or station A. If it were a single loop, like circuit 4, Fig. 21, then there would be only one sending apparatus and one receiving and transferring apparatus at the intermediate stations.
A telephone circuit will include all stations, in order to give orders to the station attendants and to signal to the central station in case of an accident, when it might be necessary to stop the air-compressor. The telephone wires,79 in the form of a lead-covered cable, are laid in the same trench with the tubes and fastened to them.
The Sending Apparatus.
—We have, in the preceding pages, frequently spoken of the sending apparatus, and have described it as mechanism by which carriers are inserted into the tube. In the Philadelphia postal line this apparatus consisted of a large valve, operated by hand. For an eight-inch tube such a valve would be too large and heavy to be manually operated. Furthermore, that type of apparatus is not suited to an intermediate station, where carriers have to pass through it. To meet all of these requirements we have designed an apparatus, of which Fig. 27 is a side elevation, Fig. 28 a longitudinal section, and Fig. 29 a cross-section. Referring to the longitudinal section, Fig. 28, the sending apparatus is shown inserted into the line of a pneumatic tube, A, A. We have a movable section of tube, B, that can be swung about the large bolt, G, at the top, into and out of line with the main tube, A, A. When the section of tube B is being swung to one side, the air-current has a by-pass through the slots E and F and the U-shaped pipe D. The joints at the ends of the movable section B are packed with specially-formed leathers. Referring to the cross-section, Fig. 29, when the movable section of tube B is swung out of line with the main tube, another and similar tube, C, takes its place. The two movable tubes, B and C, are made in one piece, so that they must always move together. They are connected together at each end by plates, M, that serve not only as connecting-plates, but covers for the ends of the main-line tube while the tubes B and C are being moved. The tubes82 B and C swing between four plates or wings, L, that extend out on each side of the apparatus. They serve as guards, and, at certain positions of the swinging tubes, prevent the air from escaping.
We will, for convenience, call the system of swinging tubes B and C, with their supports, etc., the swing-frame or simply the frame. This frame is moved or swung from one position to the other by means of a cylinder and piston, H, placed in an inclined position under it. A lug, N, is cast on the tube B, to which the connecting-rod, O, is attached. The cross-head, P, slides upon an inclined guide, Q. On top of the cylinder is placed a controlling valve, made in the form of a piston slide-valve. The piston in the cylinder H is moved by the pressure of the air taken from the main tube through the pipe I. The apparatus is operated by a hand-lever, K. When this lever is pulled, it moves the sliding-head R, and this, through the spring S, moves the controlling valve, if the valve is not locked. If it is locked, pulling the lever simply compresses the spring S. When the controlling valve is moved to the right the air in the cylinder H escapes through the passage V and the port J to the atmosphere, and compressed air from the main tube flows through the pipe I, the passages T and U, to the cylinder H, under the piston, causing the piston to move up the inclined cylinder and swing the frame until the tube C is in line with the main tube. Carriers are despatched by placing them in the tube C, then pulling the lever K, and swinging the frame until the tube C is in line with the main tube. The carrier is then taken up and carried along by the current of air in the main tube.
Replacing the hand-lever K in its original position returns the frame to its normal position.
Sending Time-Lock.
—In any system of large pneumatic tubes a short time should elapse between the despatching of carriers, in order that they may not collide in the tube, and to give the receiving apparatus at the stations time to act. To insure the impossibility of having carriers despatched too rapidly, we place on the sending apparatus a time-lock that will automatically lock it for a determined length of time after each carrier is despatched, the time-lock being adjustable for any desired time. The time-lock, W, is shown attached to the sending apparatus in Fig. 27. When the swing-frame is swung to despatch a carrier, it pulls up the rod X by means of a link and bell-crank, Y, thereby locking the controlling valve of the cylinder H and starting the time-lock W, which will unlock the controlling valve after the required time has elapsed. The details of this time-lock are shown in Fig. 30. It consists of a long vertical cylinder, A, containing a piston, B, and a spiral spring, C, that tends to force the piston to the bottom of the cylinder. The cylinder is filled with oil, and holes, D, in the piston allow the oil to pass freely through it when it is moved upward in the cylinder. When the piston moves downward an annular collar, E, forming a valve, closes the holes in the piston and prevents the oil from passing through. Extending from one side of the piston to the other is a by-pass, F, in the wall of the cylinder. When the piston moves downward the displaced oil is forced to flow through this by-pass. A small cock, G, is arranged in the by-pass to throttle the stream of oil86 flowing through it. The opening in this cock, or the amount of throttling, is indicated on the outside by an index and dial, Z (see Fig. 27). When the piston B is raised and allowed to descend by the force of the spring C, it forces the oil through the by-pass F and the cock G. If the latter is wide open the piston will descend quickly, but if it is nearly closed the piston will descend very slowly. In other words, the time of descent can be regulated by opening and closing the cock G. The reading on the dial Z can be made seconds of time that elapse while the piston is descending.
Above the cylinder is a cross-head, H, that moves up and down between vertical guides. This cross-head is moved by the rod X, also shown in Fig. 27, that receives its motion from the swinging frame of the sending apparatus. A piston rod, I, attached to the piston in the cylinder, extends up through the travelling cross-head but is not attached to it. On the piston-rod are two enlargements, J and K, one made a solid part of it, the other formed by two nuts. The travelling cross-head H carries a pawl, L, that engages under the shoulder formed by the nuts K. This pawl is kept against the piston-rod by the spring M. The enlargement, J, on the piston-rod forms a shoulder that bears against the bell-crank, N, that connects with the bolt, O, which locks the controlling valve. In the present down position of the piston and piston-rod, the enlargement J, by pressing against the bell-crank N, holds the bolt O in an unlocked position. When a carrier is despatched the cross-head H is lifted by the rod X, and carries with it the piston and piston-rod, compressing the87 spring C. This upward movement of the piston-rod allows the bolt O to be thrown by a spring, not shown in the figure, and so lock the controlling valve of the sending apparatus. As the cross-head continues its upward movement, the pawl L comes in contact with the end of the screw P and disengages the piston-rod. This allows the piston to descend as rapidly as the oil can pass through the by-pass and cock G. When the piston has reached nearly to the bottom of the cylinder, the shoulder J, on the piston-rod, engages the bell-crank N and withdraws the bolt O, thereby unlocking the controlling valve. The time that the sending apparatus is locked depends upon the time required for the piston to descend. While the sending apparatus is locked against the sending of another carrier, it is not so locked that the swing-frame cannot be returned to its normal position and another carrier inserted ready to be sent as soon as the necessary time expires. This time is usually not more than ten seconds. Not only may the second carrier be placed in the tube C, Fig. 29, ready to be sent, but the handle K may be pulled and fastened in the notch a, thereby compressing the spring S, which, as soon as the controlling valve is unlocked, will move the valve and automatically despatch the carrier. The controlling valve is locked by the passage of a bolt through the hole b, in a block carried on the end of the valve stem, when it returns to the normal position shown in the figure. Usually little or no time will be lost in thus locking the sending apparatus, for the small amount of time that the apparatus is locked will be needed in handling the carriers.
Intermediate Station Time-Lock.
—We have another time-lock attached to the sending apparatus that has been already referred to in describing the “block system” used at intermediate stations; a time-lock to prevent carriers being inserted into the tube at intermediate stations while another carrier is passing that station. This time-lock is shown in Fig. 27 at W´, and is shown in detail by a sectional drawing, Fig. 31.
When a carrier closes an electric circuit in passing one of the boxes located in a manhole about three hundred feet from an intermediate station, it indicates its approach to the station by exciting the electro-magnet A, Fig. 31. This magnet pulls down its armature B and raises the small piston valve C, which admits compressed air to a small chamber, D. The air is supplied to this chamber from the main tube through the pipe E. In one end of this chamber is fitted a piston, F, held to one end of its stroke by a spring, G. When compressed air is admitted to the chamber D, this piston is moved to the left, and by such movement throws the controlling valve of the sending apparatus into its normal position (shown in Fig. 29) and holds it there. This forms a positive lock, and, no matter in what position the sending apparatus may be, it puts the tube B, Fig. 29, into line with the main tube so that the approaching carrier can pass through the apparatus. The piston-rod H, Fig. 31, is connected to the finger d, Fig. 29, and by rocking this finger moves the controlling valve, or prevents it being moved by the handle K.
Returning now to Fig. 31, we have on the top of the90 chamber D, in addition to the electro-magnet A and its armature B, a differential cylinder and piston, K, L, whose function is to close the valve C when the chamber D is filled with air. The piston K is smaller than the piston L, and sustains a constant air-pressure, supplied through the small pipe M M, from the pipe E, which leads to the main tube. When the chamber D becomes filled from the pipe E through the valve C, the pressure in the chamber moves the piston L upward against the pressure on the piston K, because of the greater area of the piston L. This movement of the differential piston raises the lever I, which passes through a slot in the stem of the differential piston, and thus closes the valve C. The air in the chamber now gradually escapes to the atmosphere through a small orifice Q; in fact it has been escaping here all the time while the chamber was being filled, but the opening through the valve C is so many times larger than the orifice Q that the escape of air was not sufficient to prevent the chamber from filling. Now, however, that all supply to the chamber is shut off, the air in the chamber is gradually being discharged through the orifice. When nearly all the air has escaped, the piston F will return to its normal position, shown in the figure, and unlock the controlling valve. The time required for the air to escape from the chamber, D, is the time that the sending apparatus will be locked, and this time can be regulated by varying the size of the orifice Q. The opening of the orifice, or the time that the sending apparatus is locked, is indicated by an index and dial, P.
This locking mechanism is secured to a bracket on the91 side of the large cylinder H, Fig. 27, in a position where it can be easily inspected. The moving parts of the electro-magnetic valve—for such is the valve C, with the magnet A, Fig. 31—are made very light, in order that they may respond easily and quickly to the closing of the electric circuit.
It is a disadvantage to have stations too numerous upon the same line, especially if they do a large amount of business, for each station will delay the sending of carriers from the others more or less, and the interference will be greatest during the busiest hours of the day. This condition is inherent in any system of large tubes where carriers have to be run a certain minimum distance apart, and cannot be overcome by any mechanism. But the disadvantage is greatly overshadowed by the advantage of being able to connect several stations by one line, instead of having to run independent lines from each station to the central, especially when the business of the individual stations is not sufficient to occupy a separate tube all the time. It makes it possible to have stations where otherwise the business would not warrant the cost of installation and expense of operation. We recommend the establishing of not more than eight stations on a line, and usually a smaller number than this, depending, of course, upon the amount of business to be done at each station.
The Electro-Pneumatic Circuit-Closer.
—There is one piece of mechanism used in connection with the sending apparatus that we have yet to describe, and that is the circuit-closing device located in the manholes in the street. Since the carriers travel at a high rate of speed, they92 should not be made to operate any mechanism by impact with fingers or levers protruding into the tube when it can be avoided, even though the work to be done is so slight as the closing of an electric circuit, for the repeated impacts cannot fail to work injury to the carriers and the mechanism to be operated, no matter how carefully they are designed. To avoid such impacts, we have designed the electro-pneumatic circuit-closer, shown by the drawing in Fig. 32. It is operated by a passing carrier, but pneumatically rather than mechanically. In the figure we have a pneumatic tube, A, A, in which a carrier, B, is moving in the direction indicated by the arrow. At two points, about twenty or thirty feet apart, two small holes are tapped into the tube and pipes, C and D, are screwed in. These pipes lead to two chambers in a cast-iron box, F, separated by a diaphragm, E. This diaphragm is insulated electrically from the box supporting it, and is connected with the wire G. Just out of contact with the diaphragm is an insulated screw, H, connected with the wire I. These wires lead to the time-lock, already described, on the sending apparatus at the station. When no carrier is passing, the air-pressure is the same on both sides of the diaphragm, but when a carrier enters that part of the tube between the two points where the pipes C and D are connected, the equality of pressure on opposite sides of the diaphragm is destroyed. There is always a slightly greater pressure in rear of the carrier than in front of it, equal to the frictional resistance of the carrier in the tube. It is this difference of pressure in front and in rear of the carrier that moves it through the tube. When the carrier is in94 the position shown in the figure, the same difference of pressure will exist on opposite sides of the diaphragm, and it will be deflected into contact with the screw H, thereby closing the electric circuit. When the carrier has passed, equality of pressure on opposite sides of the diaphragm is established and the diaphragm takes its normal position, out of contact with the screw H. This apparatus is easily attached to the tube, and it contains no mechanism to get out of order.
The Open Receiver.
—Wherever the pressure in the tube is down nearly to atmospheric, we can use an open receiver to discharge the carriers from the tube. This is a receiver that opens the tube to the atmosphere and allows the carrier to come out. Such a receiver is used at the main post-office in the Philadelphia postal-line, and was described in the last chapter. The present receiver is similar in operation, but contains some improvements in details. Fig. 33 is a side elevation of the apparatus, Fig. 34 is a longitudinal section, and Fig. 35 is a cross-section through the cylinder and valve, showing the sluice-gate.
Referring to the longitudinal section, the apparatus is attached to the end of a pneumatic tube, A. The current of air from the tube A flows through the slots B into a pipe, C, that conducts it to a tank near the air-compressor. About the centre of the apparatus is a sluice-gate, E, that is raised and lowered by a piston in a vertical cylinder, F, located just above the sluice-gate. This piston is moved by air-pressure taken from some part of the system. When a carrier arrives from the tube A, it passes over the slots B and runs into the air-cushion D, where it comes gradually98 to rest. Checking the momentum of the carrier compresses the air in front of it considerably, and this excess of pressure is utilized to move a small slide-valve that controls the movement of the piston in the cylinder F, so that as soon as the carrier has come to rest the sluice-gate rises and allows the carrier to be pushed out with a low velocity on to a table. The small pipe G conducts a small portion of the air compressed in front of the retarded carrier to the controlling valve, H, seen in Figs. 33 and 35. Referring now to the section of the valve and cylinder, Fig. 35, the pipe G enters the top of a small valve-cylinder containing a hemispherical piston, I, that is held up by a spiral spring, J. This spring has just sufficient tension to hold the piston I up against the normal pressure of air in the tube. When a carrier arrives and compresses the air in the air-cushion, the excess of pressure forces the piston I down against the spring J, and moves the piston slide-valve K. This change of position of the slide-valve allows the air in the cylinder F to escape to the atmosphere through the passage L, passage P, and pipe M, while compressed air from some part of the main tube enters through the port N and passage O to the under side of the piston in the cylinder F. This moves the piston up, carrying with it the sluice-gate E.
There is just sufficient pressure in the tube in rear of the carrier to push the carrier past the gate and on to the table. As the carrier moves out it raises a finger, Q, Fig. 34, that projects into its path. Raising this finger extends the spring R, Fig. 33, and rotates the lever S, bringing the pawl T under the end of the controlling valve-stem.99 When the carrier has passed out and the finger Q is free to descend, the spring R rotates the lever S back to its original position, and thereby raises the controlling slide-valve, which causes the sluice-gate to close. By having the upward motion of the finger Q simply extend the spring R, and the downward motion, by the force of the spring, move the valve, we are enabled to have several carriers pass out of the tube together without having the sluice-gate close until the last carrier has passed out. If raising the finger Q moved the valve, then when the first carrier passed out, the gate would close down upon the second. Attached to the receiving apparatus and extending beyond it is a tube, U, cut away upon one side so that the carriers can roll out of it on to a table, and having in the end a buffer to stop the carriers if by any accident they come out of the tube with too much speed. This buffer consists of a piston covered with several layers of leather and having a stiff spring behind it. The whole apparatus is supported from the floor upon suitable standards, and, for an eight-inch tube, occupies a floor-space twelve feet long by two feet wide, not including the table.
This is the simplest form of receiving apparatus. Owing to conditions of pressure already explained, its use is confined principally to the pumping stations. The only care that it requires is an occasional cleaning and oiling.
The Closed Receiver.
—Next we will turn our attention to the closed receiving apparatus used at all terminal stations where the pressure in the tube is considerably above the pressure of the atmosphere, so much so that the tube cannot be opened to allow the carrier102 to pass out without an annoying blast of air and a high velocity of the carrier. This apparatus is similar to the receiver used in the sub-post-office of the Philadelphia postal line, but contains several modifications and improvements tending towards simplification. Fig. 36 shows it in elevation, and Fig. 37 in longitudinal section. As in the open receiver just described, the air from the tube A is deflected through slots B into a branch pipe, C, that conducts it from the receiving apparatus to the sending apparatus and return tube. The carriers arrive from the tube A, pass over the slots B, where the air makes its exit, and run into an air-cushion, D. This air-cushion is a tube about twice the length of the carrier, closed at one end, and supported upon trunnions. When the carrier has been brought to rest, this closed section of tube is tilted by the movement of a piston in a cylinder to an angle that allows the carrier to slide out; the tube then returns to its original position. If the end of the air-cushion was closed perfectly tight the carrier, after coming to rest, would rebound and might be caught in the joint between the stationary and movable parts of the apparatus, when the air-cushion tube tilted. To prevent the rebounding of the carrier a relief-valve, E, has been placed in the head of the air-cushion tube. It is held closed against the normal pressure in the tube by a spiral spring, but the excessive pressure created by checking the momentum of the carrier opens the valve and allows a little air to escape through the passage F and pipe G, down the pedestal H, to the atmosphere. When the air-cushion or receiving tube D is tilted to discharge a carrier,103 the circular plate I covers the end of the main tube. In order to prevent carriers sticking in the receiving tube when it is tilted, and to insure their prompt discharge, the pipe J is provided. In the tilted position of the receiving tube, the end of this pipe coincides with the end of the main tube, from which it receives air to hasten the discharge of the carrier. A check-valve, K, prevents the air from flowing backward in this pipe when a carrier is being received in the air-cushion chamber. The opening of this check-valve can be adjusted by a screw, thereby regulating the speed of ejection of the carrier.
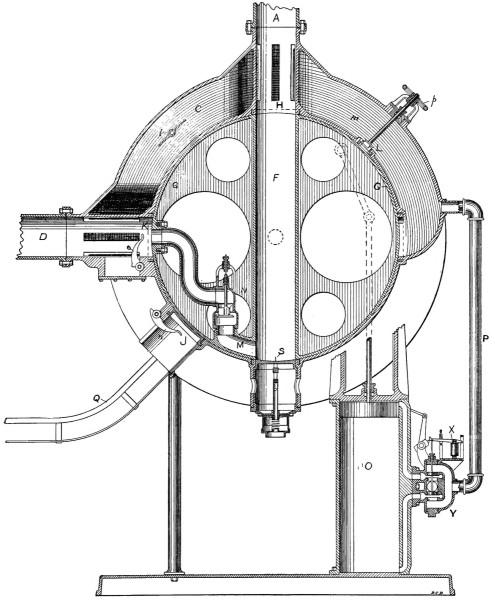
Fig. 39.
INTERMEDIATE STATION RECEIVING AND TRANSFER APPARATUS.—VERTICAL
SECTION.
Larger image (326 kB)
The carrier is discharged down a chute, L, which has a buffer at the bottom, and from the chute it rolls off on to a table. The buffer is made similar to the buffer in the open receiver already described. The cylinder and piston M, that operate to tilt the receiving tube D, are supported upon the base of the apparatus under the closed end of the receiving tube. The cross-head of the piston- and connecting-rods travels between guides that are made a part of the upper cylinder head. The movement of the piston in the cylinder M is controlled by a piston slide-valve exactly similar to the one shown in Fig. 35. The slide-valve is moved, in the same manner, by the air compressed ahead of the carrier when it is brought to rest in the air-cushion D. The air is conducted from the air-cushion to the controlling slide-valve through a small pipe, N, Fig. 36. This pipe leads to one of the trunnions, where it has a joint to allow for the tilting of the receiving tube. When the carrier is discharged from the receiving tube, it raises a finger, O, Fig. 37, located just outside the tube. Raising106 this finger pulls the rod P, Fig. 36, extends the spring Q, turns the lever R, and catches the pawl S, under the end of the controlling valve stem. When the carrier has passed down the chute and allowed the finger O to drop down, the spring Q turns the lever R back to its original position and moves the controlling valve. This causes the receiving tube to return to a horizontal position, where it is ready to receive the next carrier.
At first this apparatus may seem a little cumbersome, but nothing could work better. It is certain in its action and almost noiseless. Carriers are received, discharged, and the receiving tube returned to its normal position in four seconds, and it can be done in less time if necessary.
The Intermediate Station Receiving and Transfer Apparatus.
—One other form of receiving apparatus remains to be described, and this is the apparatus used at intermediate stations to intercept all carriers intended for that station and to send the others on through the tube to the next station. A side elevation of the apparatus is shown in Fig. 38 and a vertical section in Fig. 39. The tubes are led into an intermediate station, carried upward, and then, with a bend of one hundred and eighty degrees, are connected to the top of the receiving and transfer apparatus, as shown in the diagram, Fig. 26. The object of this arrangement will be seen as we describe the apparatus. Referring to the sectional drawing, Fig. 39, the connection of the tube A is seen at the top. As in the other receivers, the current of air arriving from the tube A is deflected through slots, B, into a passage, C, made in the frame of the apparatus. From this passage it enters the tube D through107 the slots E. The tube D leads to the sending apparatus and on to the next station, as seen in Fig. 26. The carriers are received in a closed section of tube F, which forms an air-cushion, similar to the closed receiver last described. This receiving tube F is made a part of what we might term a wheel. This wheel fits accurately into a circular casing and is supported by two trunnions or axles, upon which it revolves. The wheel has a broad flat rim, G, that covers the end of the tube at H when the wheel is revolved, and, in the normal position in which it is shown in the figure, covers the interior openings I, J, K, and L, in the casing. Leather packing is provided around each of the openings to prevent the escape of air between the face of the wheel and the interior face of the casing. From the bottom of the receiving tube F a passage, M, leads past a check-valve, N, to the tube D. When a carrier arrives from the tube A, it descends into the receiving tube F, compressing the air in front of it. This compressed air begins to escape through the passage M, but the high velocity of it closes the check-valve N as much as possible. A stop on the stem of the valve prevents it being closed entirely. The small opening past the valve allows some of the air to pass, thereby preventing the carrier from rebounding on the air-cushion. As soon as the carrier has come to rest, the check-valve N, by its own weight, opens wide, and the carrier, by its weight, settles gradually down to the bottom of the receiving tube. The wheel containing the receiving tube and the carrier will then be revolved by the cylinder and piston O, which is operated by compressed air taken from the tube through the pipe P. If the carrier108 is for this station, the wheel will rotate through an angle of forty-five degrees and discharge the carrier through the opening J, down the chute Q, from which it will roll on to a table arranged to receive it. If, however, the carrier is intended for some other station, the wheel will rotate through an angle of ninety degrees and discharge the carrier through the opening K into the tube D, and it will go on its way to the next station. This selection of carriers is brought about in a comparatively simple manner. At the bottom of the receiving tube F there are two vertical needles, R and S, shown upon a larger scale in Fig. 40. The needles R and S are contained in tubes having an insulating lining which keeps them out of electrical contact with the frame of the apparatus. Wires a and b make connection with the needles through metal plugs that form a guide for the needles, and through the springs U and V. Directly below the needle R is an insulated spring clip, W, held by two bolts and connected to the wire e.
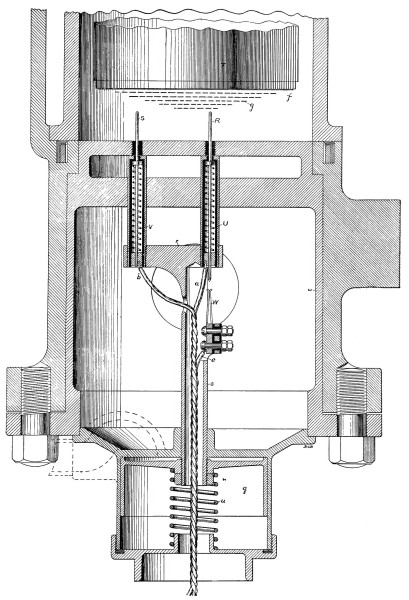
Fig. 40.
A DETAIL OF THE INTERMEDIATE STATION RECEIVING AND TRANSFER
APPARATUS.
Larger image (360 kB)
The end of a carrier is represented at T. As the carrier settles down to the bottom of the receiving tube, it comes in contact with the ends of the needles and presses them down, they being supported by two springs U and V. As the needle R is moved down, it makes contact with the spring clip W, located just below it, and closes an electric circuit that includes the electro-magnet X, Figs. 38 and 39, on the valve of the rotating cylinder O. When this electro-magnet is excited it attracts its armature and moves the piston slide-valve Y, that admits air to the top of the piston in the cylinder O, and allows the air under the piston to escape to the atmosphere. The piston moves110 downward and revolves the wheel by means of a connecting rod.
Upon the end of the carrier T is placed a thin circular metal disk, f, which may be copper, brass, tin-plate or any metal that is not easily oxidized. The diameter of this disk of metal determines the station at which the carrier will be discharged from the tube. Disks of various diameters, that may be attached to the carrier, are represented by dashed lines, g, in Fig. 40. When the carrier comes in contact with the two needles R and S, if the circular metal disk on the front end of the carrier has a diameter sufficient to span the space between the two needles, in the position in which it is held, then an electric circuit, made by the wires a and b, will be closed through the needles and the metal disk on the carrier. The metal disk makes a short-circuit from one needle to the other. If the metal disk is not large enough to span the distance between the two needles, then the electric circuit remains broken.
Returning again to Fig. 39, we have the opening J, where the carriers are discharged, closed by a sluice-gate. This gate is opened and closed by a piston moving in a cylinder, h, shown in Fig. 38. A piston slide-valve, i, similar in all respects to the valve on the cylinder O, controls the movement of the piston in this cylinder and the sluice-gate to which it is attached. The slide-valve is moved in one direction, that opens the sluice-gate, by an electro-magnet in the circuit of the wires a and b, Fig. 40.
When the electric circuit made by these wires is closed by a disk on the front end of a carrier, short-circuiting the111 two needles, the valve is moved by the electro-magnet in the circuit, and the sluice-gate is opened. As the wheel, including the receiving tube and carrier, revolves, a lug, j, Fig. 38, on the outside of the wheel comes in contact with the open sluice-gate and the wheel can rotate no farther. A blast of air through the valve L, Fig. 39, assisted by gravity, pushes the carrier out of the receiving tube, through the opening J and down the chute Q, on to the receiving table.
Had the disk on the front end of the carrier been too small to span the distance between the two needles, the circuit would not have been closed, the sluice-gate would not have been opened, no obstruction would have been placed in the path of the lug j, on the wheel, and the wheel would have continued its rotation through ninety degrees until the receiving tube F came in line with the tube D. During the latter part of the rotation, a pin on the wheel engages a lever, k, Fig. 38, and turns a valve, l, Fig. 39, stopping the flow of air through the passage C, compelling it to take another route through the passage m, and the receiving tube F, taking with it the carrier into the tube D. When the carrier leaves the receiving tube and passes through either of the openings J or K, it engages one of the fingers, n or o, that lie in its path. These fingers are connected by rods and levers to the valves on the rotating and sluice-gate cylinders. The ejected carrier pushes these fingers to one side, and after it has passed the fingers return, by the force of a spring, to their former position and move the valves, causing the sluice-gate to close and the wheel to rotate backward into its normal112 position ready to receive the next carrier. The connection between the fingers and the valves is similar to the mechanism on the open and closed receivers, so need not be described in detail here.
The speed with which the carriers are ejected from the receiving tube through the opening J and down the chute Q is regulated by the valve L, which can be opened or closed by a hand-wheel, p. Before the wheel and receiving tube can be rotated, the needles must be withdrawn from the receiving tube, and this is accomplished by a small cylinder and piston, q, shown in Fig. 40. The needles and their encasement are attached to a cross-head, r, on the end of a hollow piston-rod, s. When air is admitted to the top of the piston in the rotating cylinder O, Fig. 39, it is also admitted through the pipe t, Fig. 38, to the cylinder and upper side of the piston q, Fig. 40. This moves the piston q down against the force of a spring, u, and withdraws the needles from the receiving tube. This takes place after the needles have served their purpose and before the wheel is rotated. The piston q has much less inertia than the wheel, therefore it moves much quicker. When the wheel begins to rotate it closes a valve, v, in the pipe t, Fig. 38, confining the air in the cylinder q, and preventing the needles from being raised by the spring u before the wheel returns to its normal position. If by any accident the needles should be raised, no serious harm would result, for their ends would simply bear against the face of the wheel. If this took place constantly, grooves might be worn in the face of the wheel; for this reason the valve v is provided.
In order to facilitate the inspection of the needles and113 electric contact springs W, they are contained in a cylindrical brass case, w, that is held in place beneath the receiving tube by two bolts. By removing the nuts from these bolts the entire mechanism can be removed, examined, and cleaned. It also gives easy access to the receiving tube. The receiving tube is long enough to receive two carriers, if it should ever happen that two arrive at the same time.
To show how the apparatus at the various stations is arranged to correspond with the disks of various sizes attached to the front of the carriers, a diagram, Fig. 41, has been made, in which the needles at the bottom of the receiving tubes of the apparatus at six intermediate stations are represented at A, B, C, D, E, and F. Six disks of different sizes are represented at a, b, c, d, e, and f. The needles are placed farthest apart at station A and nearer together at each succeeding station until we arrive at station F, where they are nearest together. If we wish to send a carrier to station A from the central, we place the largest disk, a, upon the front end of it. When it arrives at station A, it closes the electric circuit between the needles and is discharged from the tube. Should we wish to send a carrier to station D, then we place the disk d upon the front end of it. When the carrier arrives at the station A, the disk is not large enough to span the needles; therefore the sluice-gate is not opened and the carrier is sent on in the tube. When it arrives at stations B and C, the same thing occurs again, but when it reaches station D, the needles are sufficiently close together so that the disk makes an electric circuit between them, and the carrier is discharged from115 the tube, as was intended when despatched. Since the carriers always travel in the same direction in a tube, the first station at which they arrive where the needles are near enough together to have both touch the disk, will be the station at which the carrier was intended to stop. Carriers can be despatched from any station, but if we wish to send from say D to A, they must either travel around a loop or be sent through a return tube in which the needles are arranged in the reverse order. If no disk is placed on the carrier, it will go to the last station on the line.
There are other attachments that might be made to the front end of the carriers in order to have them stop at any desired station along a line. We have worked out two other systems which are entirely mechanical in their operation, not using electric circuits and electro-magnets to move the valves. While such a mechanical system has some advantages over the present combined mechanical and electrical system, yet there is one great advantage in the latter, and that is the simplicity of the attachment made to the carrier. A round flat disk of tin-plate is attached to the front end; it is something that is not in the way; it does not prevent standing the carriers on end in racks to fill them; it is not easily injured, and only those who have had experience can realize the rough usage that the carriers receive; it is quickly and easily attached to the carrier, and it is so cheap that when bent it can be thrown away.
Carriers.
—The carriers are similar in all respects to those used in the Philadelphia postal-line, that have been described in the preceding chapter and illustrated in Figs. 18, 19, and 20. When there are intermediate stations upon116 the lines, means are provided for attaching disks to the front end of the carriers. The disks have a central stem that secures them to the bolt in the centre of the head, and are so arranged that they can be quickly attached or removed.
Many experiments have been made to find the best material for bearing-rings, but thus far nothing better than a specially-prepared woven fabric has been found. These rings will run about a thousand miles, when they become so reduced in diameter that they have to be replaced by new ones.
The most essential elements of a carrier are strength, lightness, and security of the contents. Aluminum has frequently been proposed as a suitable material for the bodies of carriers, but for the same weight steel is much stronger, especially in thin rolled sheets, and for this reason it has been used.
One of the most perplexing problems that presented itself in working out the details of the system was to design a secure and reliable lock for the lids of the carriers. We believe that the one which has been adopted fulfils all requirements in a satisfactory manner.
Some experiments have been made with carriers that open on the side, but structurally they are weak and unsuited to stand the blows that carriers frequently receive. They are not so easily and quickly filled and emptied as those that open on the end. These remarks apply to carriers for large tubes. In small tubes for the transportation of cash in retail stores, carriers with side openings are found convenient.
When United States mail is sent through tubes not used exclusively for postal service, carriers with special locks can be used, so that they can be opened only by post-office employees.
Air Supply.
—This completes the description of the special apparatus used in this system, but we have yet to say something regarding the machines that supply the air. In Paris the water from the city mains has been used to compress or exhaust the air used in small tubes, but to operate large tubes in most of our cities steam is the only available power. Except in isolated cases, an independent steam plant will be erected to supply the air for a system of tubes. This plant should be designed with a view to obtaining the maximum economy in coal consumption, labor, water, cartage, and incidental expenses. We might say that the same general rules of economy which govern the design and construction of electric-lighting plants should be applied to the plans and construction of air-compressing plants.
Three types of blowing machines are used,—viz., centrifugal fans, positive blowers, and air-compressors.
Fans.
—Very large tubes of moderate length can be operated by ordinary centrifugal fans. These fans are capable of supplying air under a pressure not exceeding ten or twelve ounces per square inch with very good efficiency. They are the simplest and most inexpensive of all blowing-machines.
Blowers.
—When tubes have a length and diameter that require a pressure from one to four pounds per square inch, some form of positive blower of the Root type can118 be used with economy. Their construction is familiar to nearly every one at all interested in machinery, so we need give no space to their description here.
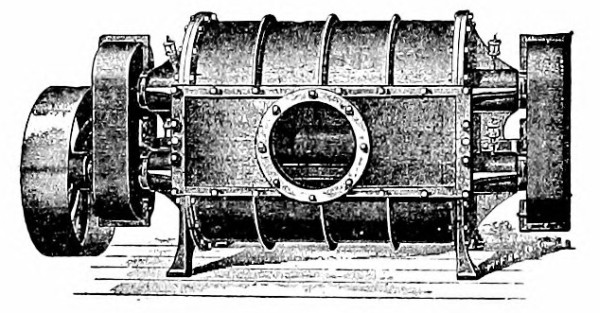
Fig. 43.
ROOT’S POSITIVE PRESSURE BLOWER.
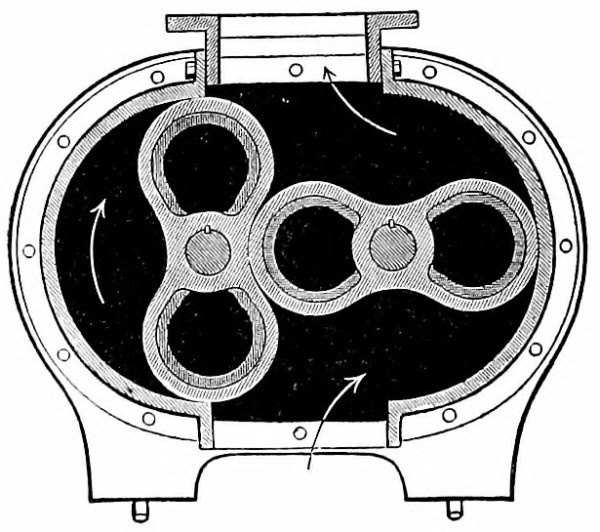
Fig. 44.
SECTION OF ROOT’S TRUE CIRCLE BLOWER.
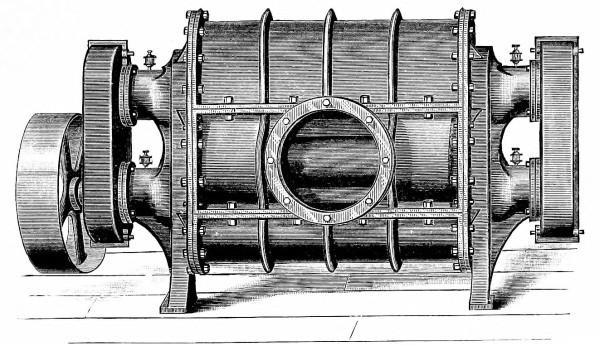
Fig. 45.
THE GREEN BLOWER.
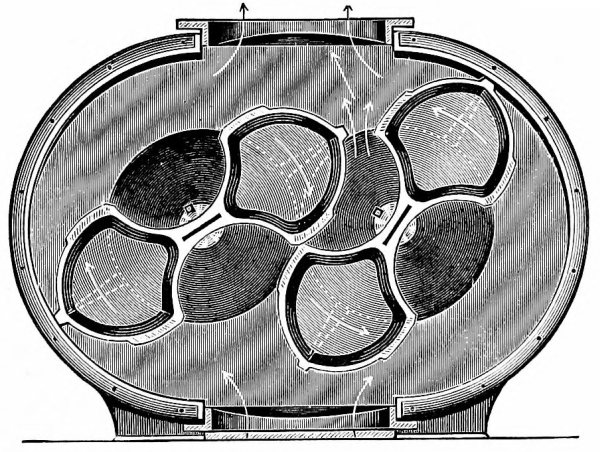
Fig. 46.
SECTION OF THE GREEN BLOWER.
Air-Compressors.
—By far the greater number of our tubes require an air-pressure of more than five pounds per square inch. For such air supply we recommend some form of air-compressor, and usually this is driven by a steam-engine, which forms a part of the compressor. In making our selection we should bear in mind the conditions under which the compressor will run. Usually it must be kept in constant operation at least ten hours per day, and frequently for a much longer period. This makes it important that the compressor be substantially built and supported upon a solid and firm foundation. The bearings should be broad, of good wearing material that has a low coefficient of friction, and provided at all times with ample lubrication. If poppet valves are used in the air-cylinders, and they are most common, the speed in revolutions per minute should not be high. Duplex are better than single cylinder compressors, because they deliver the air in a more steady stream,—the pulsations are less. For constant running, economy of steam is an important item; therefore some good type of cut-off valve should be provided. The air-cylinders should not be water-jacketed unless the pressure is above twenty-five pounds per square inch. It is better to use the air as warm as possible, for it will soon be cooled after entering the tube. A speed-governor should be provided with compressors which are to run at constant speed, but usually they will be run to maintain a constant pressure in the tank,119 and to this end a good and reliable form of pressure-governor should be provided, together with some reliable safety device to stop the engine when the speed exceeds a safe limit. But most important of all is to have the valves of the air-cylinders large in area; otherwise the efficiency of the machine will be very low. With machines working under eighty pounds pressure, a difference in pressure of one pound on opposite sides of the valves has but little effect, but when the machine is only compressing to five or ten pounds, one pound is a very large proportion of the total pressure and reduces the efficiency. Besides these few suggestions, only the requirements122 of good engineering need be demanded. In Figs. 42, 43, 44, 45, 46, and 47 we show a fan, two blowers, and an air-compressor suited to the requirements of pneumatic-tube service that can be found in the market, and that are built by responsible concerns. We believe they are all good of their kind, but do not recommend any particular make.
The Tube, Line Construction, etc.
—Up to the present time we have found no material better suited for the straight parts of pneumatic tubes than cast iron, machined upon the interior. It gives a smooth and accurate tube. It can be made in most convenient lengths. It is strong and not easily deformed. The bell-joint, calked with lead and oakum, having the tubes fitted together male and female at the bottom of the bell, is the best joint yet devised for pneumatic tubes. It is slightly yielding, accommodating itself to slight changes of length of tube due to changes of temperature, and it allows slight bends to be made at each joint. The joints are very accurate, presenting no shoulders to obstruct the passage of carriers. The joints can be made by men accustomed to laying water- and gas-pipe. The cast iron is so stiff that it is not distorted in calking, as may be done with wrought-iron tube. The principal objections to its use are the expense of boring and the readiness with which it corrodes upon the interior.
We are always hoping that wrought-iron or steel tubes will be so much improved in uniformity of dimensions and smoothness of interior that we can use them, but our experiments thus far have been discouraging. It may be123 that some of the new processes of making tubes will give us what we want, but we have not yet found it.
Small tubes and the short bends of large tubes are made of brass, it being the most suitable material. It would be very difficult to bend iron tubes without involving great expense. The thickness of the bent portion of an eight-inch tube is usually three-sixteenths of an inch and never less than one-eighth of an inch.
Where the ground is firm, no other support is needed for the tubes than to tamp the earth solidly about them. In order to economize space in the streets, it is customary to lay the tubes one above the other; and it is very convenient, although not necessary, to separate them by cast-iron saddle brackets. Such an arrangement has to be frequently departed from in order to overcome obstructions in the streets and to get through narrow passages. At all low points in a tube line, traps are provided to catch any moisture that may accumulate. These traps are made accessible for frequent inspection by means of man-holes or otherwise. The tube is usually laid about three feet below the pavement. This distance has frequently to be varied, but it never becomes so small as to render the tubes liable to injury from heavy trucks passing over the pavement.
CHAPTER IV.
FACTS AND GENERAL INFORMATION RELATING TO PNEUMATIC TUBES.
We will now discuss, in an elementary way, the theory of pneumatic tubes, in order to understand more clearly their modus operandi and the principles upon which they should be constructed. Let us begin with the definition of a pneumatic tube.
Definitions.
—A pneumatic tube is a tube containing air. This is perhaps the broadest and most comprehensive definition that can be given, but we usually associate with the idea of a pneumatic tube the use to which it is put. If we were to embody this idea in our definition we might define a pneumatic tube as a tube through which material is sent by means of a current of air. This is still a very broad definition, including all kinds of material for transportation, for every conceivable purpose. It places no limit upon the dimensions of the tube nor the manner of its operation. This definition would include the toy commonly known as a putty-blower, and the pneumatic gun.
These instruments are not usually pictured in our minds when we hear or see the term pneumatic tube used. Instead of these, we think of the brass tubes that we have seen in the large retail stores in some of our cities for conveying cash from the various counters to the centrally located cashier’s desk. Again narrowing our definition to125 conform more nearly with the mental picture presented, we will define a pneumatic tube as a long tube for the purpose of transporting material in carriers by means of a current of air in the tube. This, like all definitions, is not entirely satisfactory, if we examine it critically, but it will answer our present purpose.
Intermittent and Constant Air-Current.
—Having thus defined a pneumatic tube, there are two ways in which we may operate it to transport our carriers containing mail, packages, or other matter. The first method consists in storing our compressed air in a suitable tank, or by exhausting the air from the tank; then, when we wish to despatch a carrier we place it in the tube and connect the tube with the tank by opening a valve. As soon as the carrier arrives at the distant end of the tube the valve is closed and the air soon ceases to flow. When a long interval of time elapses between the despatching of carriers, this is the most economical method of operation, but usually carriers have to be despatched so frequently that a great deal of time would be lost if the air-current had to be started and stopped for each carrier.
The second and more usual method of operation consists in maintaining a constant current of air in the tube and in having the carriers inserted and ejected at the ends of the tube without stopping the current of air for any appreciable length of time. It is analogous to launching boats in a rapidly flowing stream, allowing them to float down stream and then withdrawing them. When the boats are in the stream they present little obstruction to the flow of water and check its speed but very little. In order to126 compute the speed with which the boat will pass from one point to another, we only have to know the speed of the stream between those points when no boat is in it. The presence of the boat does not change the speed appreciably. So it is with carriers in a pneumatic tube: they are carried along with the current of air. The air flows nearly as rapidly when a carrier is in the tube as when there is none. The friction of the carrier against the inner surface of the tube creates a slight drag, but it checks the speed of the air only a little. Therefore, in order to know the speed with which a carrier will be transported from one station to another through a pneumatic tube, we need only to know the velocity with which the air flows through the tube when no carrier is present. Of course there are special cases of heavy carriers, or carriers having a large amount of friction from their packing, or of tubes not laid horizontally, where the resistance of the carrier must be taken into consideration, but for our present purpose we will neglect all of these conditions.
Laws Governing the Flow of Air in Long Tubes.
—This leads us to study the laws governing the flow of air in long tubes, omitting for the present the presence of a carrier. Since tubes operated intermittently have become obsolete, we will only consider the case of a constant current of air, this being what we have to deal with in practice.
In order to make our ideas and thoughts as clear as possible let us represent them by a diagram, Fig. 48. We will suppose that a tank, A, is kept constantly filled with compressed air at a pressure of ten pounds per square128 inch, from some source of supply. We will suppose that the pressure of the air in this tank never changes, air being supplied as fast as it flows away. Next, let us assume that a tube eight inches in diameter inside and one mile long (five thousand two hundred and eighty feet) is connected to the tank at one end and left open to the atmosphere at the other. The air will flow in a constant stream from the tank into the atmosphere, for the reason that air is being supplied to the tank as fast as it flows away.
Law of Pressure.
—First, let us consider the pressure of the air at various points in the tube. We will, for convenience, represent the pressure in the tank by a vertical line, D E, ten units in length, since the pressure is ten pounds per square inch. Now let us go to a point on the tube one quarter of a mile (one thousand three hundred and twenty feet) from the tank, drill a hole in the tube, attach a pressure-gauge and measure the pressure of the air at this point. We shall find it to be about 7.91 pounds per square inch; or, 2.09 pounds below the pressure in the tank. We will represent this on our diagram by another vertical line, F G, having a length of 7.91 units. Again let us measure the pressure in the tube at a point one-half a mile (two thousand six hundred and forty feet) from the tank. Here we find it to be about 5.61 pounds per square inch, and we represent it by the vertical line, H I, having 5.61 units of length. We note that the pressure is 4.39 pounds below the pressure in the tank. We are at the middle point of the tube and the pressure has fallen to nearly, but not quite, one-half the pressure in the tank. We will now go to a point three-quarters of a mile (three thousand nine129 hundred and sixty feet) from the tank, and here the pressure is about 3.01 pounds per square inch. We represent it by the vertical line, J K. Lastly, we measure the pressure very near the end of the tube, one mile from the tank, and find it to be about zero, or the same as the pressure of the atmosphere. All of our measurements have been in pounds above the atmospheric pressure; to express them in absolute pressure, we should add to each the pressure of the atmosphere, which is 14.69 pounds, nearly.
Now we will draw a smooth curve through the tops of all our vertical lines, and we have a curve, E, G, I, K, L, representing the pressure in the tube at every point. It falls gradually from ten pounds to zero, but it does not fall in exact proportion to the distance from the tank. Such a fall of pressure would be represented by the straight dash-line, E, L. The reason why the true pressure-curve is not a straight line, and lies above a straight line, is because air is an elastic fluid and expands, becoming larger in volume as the pressure diminishes. The straight dash-line represents the fall of pressure of an inelastic fluid, like water, when flowing in the tube.
The fall of pressure along the tube is analogous to the fall of level along a flowing stream. In fact, we frequently speak of the descent of a stream as the “head of water” when it is used for power purposes, and we mean by this the pressure the water would exert if it were confined in a pipe. The descent, or change of level, in the bed of a stream is necessary to keep the water flowing against the friction of the banks. The descent of the water imparts130 energy to overcome the friction. In a similar manner, we must have a fall of pressure along the pneumatic tube to overcome the friction of the air against the interior surface of the tube. We find another analogue in the flow of the electric current along a wire; here there is a fall of potential necessary to overcome the resistance of the wire. Since power has to be expended to compress the air and impart to it its pressure, when this pressure disappears we know that the air must be losing its energy or doing work, and we look to see what becomes of it. In the present case, we find that most of this work is expended in overcoming the friction between the air and the surface of the tube.
Uses of Pressure Curves.
—The pressure curve teaches us many things. Suppose we were to establish stations on this tube at the quarter, half, three-quarter, and mile points; we see at once that intermediate-station or closed receivers, described in the last chapter, must be used at all of the stations except the mile point at the end of the tube, because the pressure in the tube is so high above the pressure of the atmosphere that we could not open the tube to let the carriers come out, but at the end of the tube we could use the open receiver. In designing our sending and receiving apparatus for each station, we look to this pressure curve to tell us the pressure which we shall have on the pistons in our cylinders, and are thereby enabled to make them with proper proportions for the work that they have to do.
Law of Velocity.
—Next let us see what the velocity of the air is in the tube. Suppose that we have some convenient means of measuring the velocity of the air at any131 point, in feet per second or miles per hour, with some form of anemometer. We will have our measurements taken at the five points where we measured the pressure,—viz., at the tank, one-quarter, one-half, three-quarters and one mile from the tank. We will represent the velocities by a diagram similar to the one used for pressures. At the tank we find the air entering the tube with a velocity of 59.5 feet per second (40.6 miles per hour). We draw the vertical line M N, to represent this. At the quarter mile point the velocity is sixty-five feet per second (44.4 miles per hour) an increase in the first quarter of a mile of 5.5 feet per second. We construct the vertical line O P. At the half-mile point the velocity is 72.4 feet per second (49.4 miles per hour); at the three-quarter mile point it is eighty-three feet per second (56.8 miles per hour); and at the end of the tube, one mile from the tank, the air comes out of the tube with a velocity of 100.4 feet per second (68.5 miles per hour), about 1.7 times faster than it entered the tube at the tank. Drawing all the vertical lines to represent these velocities, and drawing a smooth curve line through the tops of our vertical lines, we have the curve of velocities, N, P, R, T, V, for all points along the tube. It is an increasing velocity and increases more rapidly as we approach the end of the tube. This is shown more clearly by drawing the straight dashed line N V.
If the fluid flowing in the tube were inelastic, like water, then the curve of velocities would be a straight horizontal line, for the water would not come out of the tube any faster than it went in. But we are dealing with air, which is an elastic fluid, and, as we stated before, it expands as132 the pressure is reduced and becomes larger in volume. It is this expansion that increases its velocity as it flows along the tube. It must go faster and faster to make room to expand. Since the same actual quantity of air in pounds must come out of the tube each minute as enters the tube at the other end in the same time, to prevent an accumulation of air in the tube, and since it increases in volume as it flows through the tube, it follows that its velocity must increase.
Characteristics of the Velocity Curve.
—This velocity curve is both interesting and surprising, if we have not given the subject any previous thought. It might occur to us that the air expands in volume in the tube, and we might reason from this fact that the velocity of the air would increase as it flowed through the tube, but very few of us would be able to see that the rate of increase of velocity also increases. That is to say, it gains in velocity more rapidly as it approaches the open end of the tube. If the velocity were represented on the diagram by a straight horizontal line, we should know that it was constant in all parts of the tube, which would be the case if water were flowing instead of air. If it were represented by a straight inclined line, like the dashed line N V, then we should know that the velocity increased as the air flowed along the tube, but that it increased at a uniform rate. The slope of the line would indicate the rate of increase. Neither of these suppositions represent correctly the velocity of the air at all points in the tube; this can only be done by a curved line such as we have shown. The slope of the curve at any point represents133 the rate of increase of velocity of the air at that point. If the curve is nearly horizontal, then we know that the velocity does not increase much; but if the curve is steep, then we know that it is increasing rapidly, the actual velocity being indicated by the vertical height of the curve above the horizontal line M U.
Use of Velocity Curves.
—Besides being interesting, a knowledge of the velocity of the air at all points in a tube is of much practical value. It gives us the time a carrier will take in going from one station to another. Usually the first questions asked, when it is proposed to lay a pneumatic tube from station A to station B, are, How quickly can you send a carrier between these points? How much time can be saved? These questions are answered by constructing a velocity curve. Since the velocity changes at every point along a tube, to get the time of transit between two points we must know the average velocity of the air between those points. We can find this approximately from our curve by measuring the height of the curve above the horizontal line M U at a large number of points, and then taking the average of all these heights; but there is a more exact and easier method by means of a mathematical formula. As such formula would be out of place here, we will not give it; suffice it to say, that the average velocity of the air between the tank and the end of the tube, in the case we have assumed, is about seventy-three feet per second (49.7 miles per hour), a little less than one-half the sum of the velocities at the two ends, and a little more than the velocity at the half-mile point. Knowing the average velocity, we can tell how134 long it takes for a particle of air, and it will be nearly the same for a carrier, to travel from the tank to the end of the tube, by dividing the distance in feet by the average velocity in feet per second. This we find to be one minute 12.3 seconds. Since the air moves more rapidly as it approaches the open end of the tube, a carrier will consume a greater period of time in going from the tank to the quarter mile point than in going from the three-quarter mile point to the open end. The last quarter of a mile will be covered in a little more than fourteen seconds, while the first quarter will require a little more than twenty-one seconds. This difference is surprising, and it becomes even more marked in very long tubes with high initial pressures. This explains why the service between stations located near the end of the tube is more rapid than between stations on other parts of the line.
This velocity curve shows us the velocity of the carriers at each station along the line and enables us to regulate our time-locks and to locate the man-holes and circuit-closers connected with each intermediate station. It gives us the length of the “blocks” in our “block system.” When we know the velocity and weight of our carriers, we can compute the energy stored up in them, and from this the length we need to make our air-cushions so as not to have the air too highly compressed. It would be impossible to design our apparatus properly if we did not know the laws that govern the flow of air in the tubes.
Quantity of Air Used.
—The next important fact that we learn from the velocity curve is the quantity of air that flows through the tube each second or minute. If we135 multiply the velocity with which the air escapes from the open end of the tube by the area of the end of the tube in square feet, we have the number of cubic feet of air at atmospheric pressure discharged from the tube per unit of time. The same quantity of air must be supplied to the tank in order to maintain a constant flow in the tube. In the present case that we have assumed, the tube is eight inches in diameter; therefore the cross-sectional area is 0.349 square foot. The velocity of the air as it comes out of the end of the tube is 100.4 feet per second; therefore about thirty-five cubic feet of air are discharged from the tube each second, or two thousand one hundred cubic feet per minute. This same amount must be supplied to the tank A in order to maintain the pressure constant, but when it is compressed so that it exerts a pressure of ten pounds per square inch, the two thousand one hundred cubic feet will only occupy a space of one thousand two hundred and fifty cubic feet, if its temperature does not change. This leads us to consider the effect of temperature changes.
Temperature of the Air.
—If the air is allowed to become heated by compression, as is the case in practice, we have a new set of conditions. If the air in the tank A is hot,—that is, warmer than the surrounding atmosphere,—it will by radiation cool somewhat before it enters the tube, and it will be still further cooled when it expands in the tube. Again, if its temperature falls below the temperature of the ground in which the tube is laid, it will absorb heat from the ground, and this will tend to keep up its temperature; so in practice we have very complicated relations between the temperature, pressure, and volume136 of the air. These relations cannot be exactly expressed by mathematical formulæ, and we will make no attempt so to express them, but will be content with saying that in practice we find that the temperature of the air in the tubes is nearly constant after the first few hundred feet, so that we can without appreciable error compute the pressures and velocities as if it were constant. Now, if the air in the tank A is hot, we must raise the pressure a little above ten pounds per square inch to obtain the velocities given on our diagram. When the air cools it contracts in volume, or, if the volume cannot change, being fixed by the limits of the containing vessel, then the pressure is reduced, so by raising the pressure in the tank A a little above ten pounds, we compensate the loss of pressure.
Horse-Power.
—Having shown how the quantity of air can be computed we are now in a position to estimate the horse-power of the air-compressor necessary to operate the tube. I say estimate, because we have to take into consideration the efficiency of the air-compressor, and that is not an absolutely fixed quantity: it varies with different types of machines and with their construction. When working with pressures of less than ten pounds, the friction of the machine is an important factor. The area and construction of the valves in the air-cylinders is another very important factor. If the valves are not large enough or do not open promptly, our cylinders will not be filled with air at each stroke; this will reduce the efficiency of the machine. In practice we go to a manufacturer of air-compressors and tell him how much air must be compressed per minute, and the pressure to which it must be compressed,137 with other conditions, and then he tells us what size of machine we shall require and the horse-power of the machine approximately. He is supposed to know the efficiency of his own machines. We may endeavor to prove his estimate by computations of our own. To give some idea of the horse-power required to supply the air needed to operate our eight-inch tube one mile long, I will say that the steam-engines of the air-compressor will have to develop in the vicinity of one hundred and twenty-five actual horse-power. From the horse-power of the steam-engine we can easily compute the coal that will be consumed under boilers of the usual type.
Efficiency.
—It will be noted that most of the power is not used primarily in moving the carriers, but to move the air through the tube. Very nearly as much power is used to keep the air flowing in the tube when no carriers are in it as when carriers are being despatched. If we should define the efficiency of a pneumatic tube as the ratio of the power consumed in moving the carriers to the power consumed in moving the carriers and the air, we should find this so-called efficiency to be very low. It is analogous to pulling the carrier with a long rope and dragging the rope on the ground. Much more power would be consumed by the rope than by the carrier. But a business man would define the efficiency of a pneumatic tube as the ratio of the cost of transporting his letters, parcels, etc., to the cost of transporting them with equal speed in any other way. Defined in this practical manner the efficiency of a pneumatic tube is high. We do not care what becomes of the power so long as it accomplishes our purpose.
Pressure and Exhaust Systems.
—We have noticed that pneumatic tubes have not always been operated by compression of the air, but that some of the small tubes used in the telegraph service of European cities have been operated by exhausting the air. The two systems are sometimes distinguished by calling one a pressure system and the other an exhaust system. These terms are very misleading, for an exhaust system is a pressure system. The current of air is kept flowing in a tube by maintaining a difference of pressure at the two ends, and the result is the same whether we raise the pressure at one end above the atmospheric or lower it at the other below the atmospheric. In either case it is pressure that causes the air to flow. It happens that we are living in an atmosphere of about fifteen pounds pressure per square inch, and it is very convenient oftentimes in our computations to take the pressure of the atmosphere as our zero, and reckon all other pressures above and below this. If all our pressure scales read from absolute zero, the pressure of a perfect vacuum, then all this confusion would be avoided. We have not used the absolute zero in our diagram, because all our gauges are graduated with their zero at atmospheric pressure, and it is customary to speak of pressures above and below the atmospheric.
It is very natural to ask the question, why are tubes sometimes operated by compressing the air and at other times by exhausting it? We answer by saying that it is usually a question of simplicity and convenience that determines which system shall be used. Some of the cash systems in the stores use compressed air in the out-going139 tubes from the cashier’s desk and exhaust the air from the return tubes. Both ends of the tubes are then left open at the counters, no sending or receiving apparatus being required there. The carriers are so light, their velocity so low, and the air-pressure varies so little from the pressure of the atmosphere that the carriers can be allowed to drop out of the tubes on to the counters, and they can be despatched by simply placing them at the open end of the tube into which the air is flowing. The currents of air entering and leaving the tubes are not so strong as to cause any special annoyance. At the cashier’s desk some simple receiving and sending apparatus has to be used, but it is better to concentrate all of the apparatus at one point rather than have it distributed about the store, as would be the case if the double system were not used. In the London pneumatic telegraph both methods of operation have been in use. Double lines were laid, and the engines exhausted the air from one tube and forced it into the other. The exhausted tube was therefore used to despatch in one direction, while the other tube, operated by the compressed air, served for despatching in the opposite direction. So far as I know, both work equally well.
In operating large tubes, that is to say, tubes six or eight inches in diameter, there is an advantage in using compressed, rather than exhausted air, in the construction of the sending and receiving apparatus, especially when the tubes are very long. With an ample supply of compressed air always at hand, the air-cushions can be made shorter and more effective in bringing the carriers quickly to rest.140 With exhausted air the cushions are ineffective, and consequently must be made very long in order to stop the carrier before it strikes the closed end of the tube. This does not apply to small tubes where the carriers are so light that they can be stopped without injury by allowing them to strike solid buffers. Again, when compressed air is used, we have a larger difference in pressure between the pressure in the tube and the atmosphere to operate our mechanism by cylinders and pistons. With an exhaust system carriers are not so easily ejected from the tubes of the receiving apparatus; we could not use the simple form of open receiver. Again, if the tubes are laid in wet ground, and a leak occurs in any of the joints, water will be drawn in if air is being exhausted from the tube, while it will be kept out if compressed air is used.
In regard to the question of relative economy of the two systems, we will say that when long tubes are used, requiring high pressures, or, more strictly speaking, a large difference of pressure, to maintain the desired velocity of air-current, there seems to be some advantage in using an exhaust system. The reason is this: the friction of the air in the tube, which absorbs most of the power, increases as the air becomes heavier and more dense. When the air is exhausted from the tube, we are using a current of rarefied air, and this moves through the tube with less friction and, consequently, a higher velocity, for the same difference of pressure, than the more dense compressed air. But for short tubes that require only a small difference of pressure, this advantage becomes very small, and is overbalanced by other advantages of a compressed air system. So, taking141 everything into consideration, there is not so much to be said in favor of an exhaust system.
Laws Expressed in Mathematical Formulæ.
—While we have heretofore purposely avoided all complicated mathematical formulæ, it may not be out of place here to give a few of the more simple relations that exist between the pressure, velocity, length and diameter of the tubes, etc. In two tubes having the same diameter, with the same pressures maintained at each end, but of different lengths, the mean velocities of the air in the tubes will bear the inverse ratio to the square roots of the lengths of the tubes. This is expressed by the following proportion:
u : U :: √L : √l
u and U represent the mean velocities of the air in the two tubes and l and L the respective lengths of tubes.
A similar but direct ratio exists between the mean velocities and the diameters of the tubes, thus:
u : U :: √d : √D
This relation, however, is only approximately true for tubes differing greatly in diameter.
The relation of the pressure to other factors is not so simply expressed. For example, in two tubes of the same length and diameter, the relation between the pressures at the ends and the mean velocity of the air may be expressed as follows:
(p02 - p12)3/2(P02 - P12)3/2
u : U :: ———— : ————
(p03 - p13)(P03 - P13)
(p02 - p12)3/2(P02 - P12)3/2
u : U :: ———— : ————
(p03 - p13)(P03 - P13)
where u and U are the respective mean velocities, p0 and P0 the respective pressures at the initial ends of the tubes, and p1 and P1 the respective pressures at the final ends of the tubes,—the pressures being measured above absolute zero.
There are other relations that can be similarly expressed, but for them we must refer the reader to a mathematical treatise on the subject.
Moisture in the Tubes.
—When pressures of more than five pounds per square inch are used, it is not unusual to find some moisture on the interior of the tube and upon the outside of the carriers when they come out of the tube. It is seldom more than a slight dampness, or at most a degree of wetness equal to that seen on the outside of a pitcher of ice-water on a warm day. A slight amount of moisture in the tube is not objectionable, for it serves as a lubricant to the carriers; but when it is present in considerable quantity it becomes objectionable and even annoying. This moisture is brought into the tube with the air, and is deposited upon the walls of the tube when their temperature is sufficiently below that of the atmosphere. The atmosphere always contains more or less moisture in the state of vapor. The capacity of air for water-vapor depends upon its temperature, being greater the higher the temperature, but it is a fixed and definite quantity at any given temperature. When the air contains all the water-vapor it can hold at a certain temperature, it is said to be saturated. If it is not saturated, we express the amount that it contains in per cent. of the amount it would contain if it were saturated, and this is termed the “relative humidity.”143 For example, if the air is three-fourths saturated, we say the “relative humidity” is seventy-five; but if the temperature changes, the “relative humidity” changes also. Suppose the temperature to-day is seventy-five degrees Fahrenheit, and that the “relative humidity” is eighty, a cubic foot of air then contains 0.00107 pound of water-vapor. Now suppose this air enters a pneumatic tube and is cooled by expansion and contact with the colder walls of the tube to sixty degrees. At this temperature a cubic foot of air can contain only 0.00082 pound of water-vapor when it is saturated. Now, each cubic foot of air brought into the tube brings with it 0.00107 pound of vapor, and after it is cooled down to sixty degrees it cannot hold it all, consequently the difference, or 0.00025 pound, must be deposited in the tube. Under these conditions one hundred thousand cubic feet of air will deposit twenty-five pounds of water in the tube.
In the system of pneumatic tubes built and operated by the Batcheller Pneumatic Tube Company, the presence of a large quantity of moisture in the tube is prevented by using the same air over and over again. A little moisture may be deposited when the tube starts into operation, but the amount does not increase appreciably, as very little fresh air is admitted after starting.
Location of Obstructions in Tubes.
—In regard to the removal of obstructions in the tubes, I have had little or no experience; therefore under this heading I am satisfied to quote from the “Minutes of the Proceedings of the Institution of Civil Engineers,” London, Volume XLIII.
“Intimately connected with the working of the tubes is144 the removal of obstructions which occur from time to time, causing not unfrequently serious inconvenience and delay. The most general cause of obstruction is a stoppage of the train arising from accident to the tube, to the carriers or piston, or to the transmitting apparatus. In such cases the delay is generally very brief, it being for the most part sufficient to reverse the pressure on the train from the next station, and to drive it back to the point it started from. If one or more of the carriers break in the tube, reverse pressure is also generally sufficient to remove the obstacle; but where this fails, the point of obstruction must be ascertained. This is done by carefully observing the variations of air pressure in the reservoir when placed in connection, first with a line of known length, and then with the obstructed tube. By this means the position of the obstruction can be ascertained within one hundred feet. Or the tube may be probed with a long rod up to a length of two hundred feet. A very ingenious apparatus, by M. Ch. Bontemps, is shown in Figs. 49 and 50, and is employed to ascertain the exact position of the obstruction. It acts by the reflection of sound-waves on a rubber diaphragm. A small metal disk is cemented to the rubber, and above this is a pointed screw, D. An electric circuit is closed where the points C and D are brought in contact. To locate an obstruction a pistol is fired into the tube as shown, and the resulting wave, traversing the tube at the rate of three hundred and thirty metres a second, strikes the obstruction and is then reflected against the diaphragm, which in its turn reflects it to the obstacle, whence it returns to the diaphragm. By this means indications are marked on the146 recording cylinder, and if the interval of time between the first and second indications be recorded, the distance of the obstacle from the membrane is easily ascertained. The chronograph employed is provided with three points; the first of these is placed in a circuit, which is closed by the successive vibrations of the diaphragm; the second corresponds to an electric regulator, marking seconds on the cylinder; and the third subdivides the seconds there marked. Fig. 50 indicates a record thus made. In this case the obstacle is situated at a distance of sixty-two metres, and the vibration marks thirty-three oscillations per147 second. The interval occupied by two successive marks from the diaphragm on the paper corresponds to twelve oscillations, and the distance of the obstruction is then calculated by the following formula:
D = 0.5 × 330 × 12⁄33 = 60 metres;
so that the distance of the obstacle is recorded within two metres.
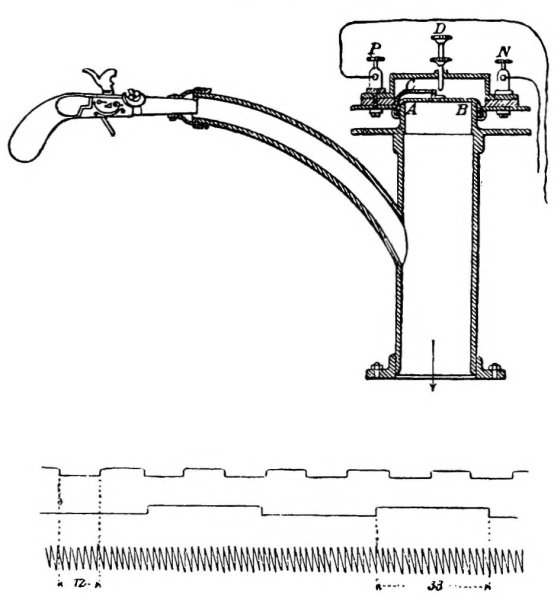
Fig. 50.
OBSTRUCTION-RECORDING APPARATUS.
“Amongst the special causes of accident may be mentioned the accidental absence of a piston to the train, breaking of the piston, the freezing up of a piston in the tube, and even forgetting the presence of a train, which has caused the entire service to be one train late throughout the day. Finally, the tubes themselves are sometimes broken or disturbed during street repairs, resulting of course in a complete cessation of traffic in the system till the damage is made good.”
Transcriber’s Notes:
The spelling, punctuation and hyphenation are as the original, with the exception of apparent typographical errors which have been corrected.